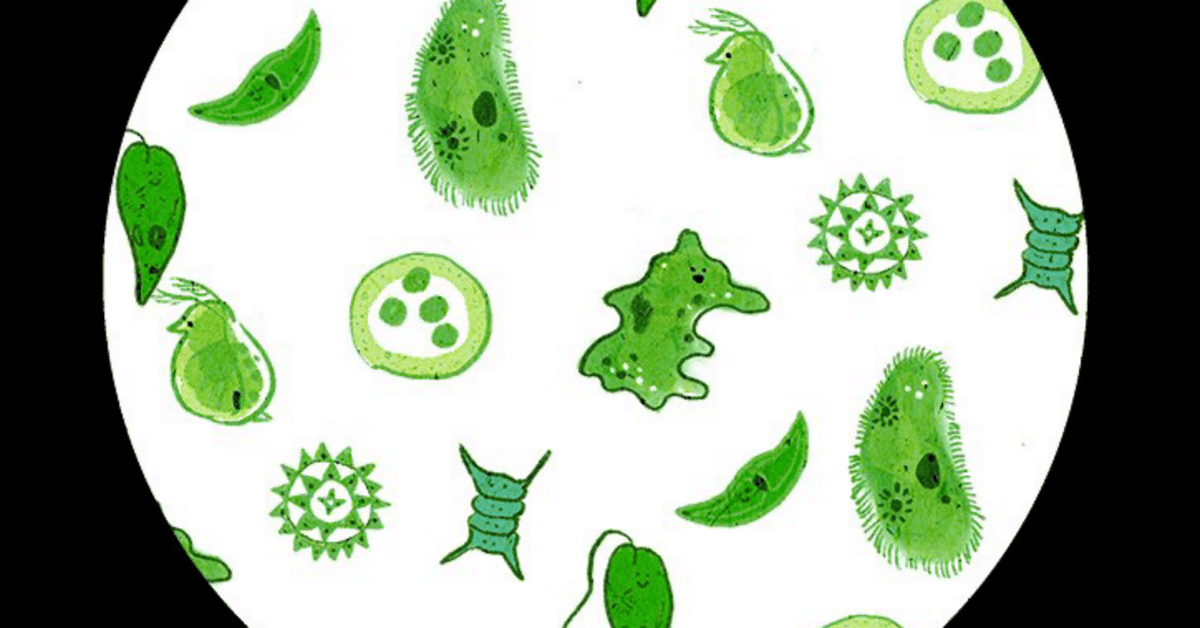
【とっても簡単に医科学トピック紹介2】見えない世界を解き明かす:メタゲノミクスの魅力
元動画はこちらです:
メタゲノミクスとは
メタゲノミクスでは、環境サンプル(例えば土壌、水、腸内など)から直接DNAを抽出し、そのDNAを解析します。この方法により、微生物の全体像をそのままの状態で解析することができます。具体的には、次のステップが含まれます:
サンプル採取: 環境からサンプルを採取します。これには土壌、水、人体の一部(例えば腸内や皮膚)などが含まれます。
DNA抽出: サンプルからDNAを抽出します。これには、微生物のDNAだけでなく、他の生物のDNAも含まれます。
シーケンシング: 抽出したDNAをシーケンシング(塩基配列決定)します。これにより、DNAの配列情報を取得します。
データ解析: 取得したシーケンスデータを解析し、微生物の種類や機能を特定します。
メタゲノミクスは、環境中の微生物コミュニティのDNAを直接解析する技術です。この分野は、特定の微生物を培養することなく、その存在と機能を解明する手段を提供します。多くの微生物は培養が難しいため、メタゲノミクスは微生物研究において非常に重要です。
メタゲノミクスの利点
培養不要: 培養が困難な微生物も解析できる。
多様性の把握: 微生物コミュニティ全体の多様性を理解できる。
機能解析: 微生物の機能や役割を特定できる。
メタゲノミクスの応用例
医療: 腸内フローラの研究や感染症の診断。
環境科学: 土壌や水中の微生物コミュニティの解析。
農業: 土壌の健康状態や作物の成長に関与する微生物の研究。
動画の概要
この動画では、メタゲノミクス技術を使って微生物のDNAを解析する方法について学びます。初めに、微生物を研究するための技術とその歴史について説明し、次にデータ分析ツールの使い方を紹介します。実際のデータセットを使った例も見ていきます。
微生物学の歴史
昔は、髪の毛の幅くらいの大きさが人間が見える最小のものでした。しかし、1673年にオランダの顕微鏡学者アントニー・ファン・レーウェンフックが顕微鏡を発明し、水や食べ物、周りのすべてに微生物が存在することを発見しました。顕微鏡の解像度が向上するにつれて、ますます多くの微生物が発見されました。今日では、非常に高性能な顕微鏡を使って、微生物の世界を詳細に観察できます。
バクテリアの培養技術
19世紀になると、バクテリアを培養するための人工的な培地が開発されました。1860年にはルイ・パスツールが初めてバクテリアを再現可能な方法で培養しました。今日でも、この方法はよく使われており、特定の培地の発見と改良によって、実験室でのバクテリアの培養と研究が進んでいます。
グラム染色
グラム染色は、バクテリアをグラム陽性とグラム陰性に分類するための技術です。バクテリアの細胞壁の物理的特性に基づいて分類され、感染が疑われる場合に迅速に結果を得ることができるため、患者の治療と予後に大きな影響を与えます。例えば、髄膜炎の診断には脳脊髄液、関節炎の診断には滑液などでグラム染色が用いられます。
メタゲノミクス
メタゲノミクスは、環境中の微生物コミュニティからDNAを直接解析する技術です。この技術により、培養できない微生物や未知の微生物を特定することができます。メタゲノミクスは、微生物の全体像を自然環境の中でそのまま解析することができるため、非常に有用です。
16SリボソームRNA
16SリボソームRNAは、メタゲノミクスでよく使われるマーカー遺伝子です。この遺伝子には種特異的な配列が含まれており、PCRによって増幅されるため、アンプリコンシーケンスと呼ばれます。16S遺伝子の配列にはハイパーバリアブル領域(HVRs)があり、これが細菌の識別に使われます。増幅された配列は、リファレンスデータベースにマッピングされ、細菌の分類に役立ちます。
OTUs(操作分類単位)
OTUsは、似たような遺伝子配列を持つ微生物のグループを表します。これらの配列は、リファレンスデータベースにマッピングされ、微生物コミュニティの割合を示します。リファレンスデータベースには、RDP(リボソームデータベースプロジェクト)、Green Genes、Silvaデータベースなどがあります。コミュニティ間や個体間の比較に使われ、データの準備方法や分析手法を理解することが重要です。
クオラムセンシング
微生物は、クオラムセンシングという方法でお互いにコミュニケーションを取ります。これは、微生物が特定の信号分子を使って、環境の変化に適応したり、病原性を発揮したりするための仕組みです。クオラムセンシングは、バイオフィルムの形成や病原菌の感染力に関与しています。
人間のマイクロバイオータ
人間の体には、10兆から100兆の微生物が存在し、これらはマイクロバイオータと呼ばれます。これらの微生物は、消化や免疫システムの発達、エネルギー生成など、さまざまな重要な機能に寄与しています。例えば、消化や栄養吸収、エネルギー生成、代謝調節、脂肪の貯蔵、環境化学物質の処理と解毒、皮膚や粘膜のバリア機能の維持、炎症プロセスの調節、病原微生物の侵入防止などです。
ヒトマイクロバイオームプロジェクト
1990年代以降、ヒト腸内細菌叢プロジェクトやヒトマイクロバイオームプロジェクトなどの大規模な研究が行われ、皮膚、肺、腸、さらには脳のマイクロバイオータが詳細に研究されるようになりました。これらのプロジェクトにより、私たちは人間の微生物コミュニティが健康や発達にどのように寄与しているかを理解するための大規模なデータセットとリソースを手に入れました。
<付録>動画の原文を書き起こしました ※視聴の際にお使いください
Introduction
Welcome to Armex Logic Metagenomics. This course is going to introduce you to bioinformatics analysis of metagenomic sequencing data. In this first session, we will start by speaking about genomic sequencing technologies as one of the methods to study microbes and map out how such data can be used. Then we will introduce you to several data analysis tools for metagenomic studies and take a look at a few examples where such analysis approaches have been used. Let's start by reviewing a little bit of history of microbiology, where the study of microorganisms led to the creation of tools and approaches that are still used today and continue to uncover the veil of a world which we do not see but yet has such a profound effect on everything around us.
History of Microbiology
For millennia, the smallest thing humans could see was about as wide as a human hair. When the microscope was invented, suddenly we saw a new world of living things in our water, our food, and in fact, everywhere around us. The first observation of a bacterium was made around 1673 by the Dutch microscopist Anton van Leeuwenhoek, thanks to the microscopes he developed. Those enlarged whatever he studied from 50 to 300 times. As the resolution of the microscope improved, more and more representatives of the unseen world have been discovered. Today, microscopes are tremendously powerful, allowing humans to see smaller and smaller elements that reveal how the world of microorganisms functions and every imaginable surface. Microbiology is defined not only by the organisms it studies but also by the actual tools used to study them. These tools have evolved to allow us to study microorganisms and categorize them. Technology for bacterial culturing, coupled with a technique to stain bacteria and view it under the microscope, has improved our ability to isolate, categorize, and determine whether a bacteria is a cause of human disease and even select an appropriate treatment. More advanced technologies like sequencing are now being used to further study the complex environments where such bacteria originate and grow.
Technology for Bacterial Culturing
Culture media allowed for the development of microbiology in the 19th century. Bacterial culture is the artificial medium that allows growth and isolation of bacteria. The first to have cultured a bacterium in a reproducible way was Louis Pasteur in 1860. Today, such methods are still often used and are being driven by discovery and perfection of specific mediums to grow and cultivate bacteria in the lab. Gram staining is a bacteriological laboratory technique used to differentiate bacterial species into two large groups, gram-positive and gram-negative, based on the physical properties of their cell walls. Gram stains are performed on body fluid or biopsy when infection is suspected. Gram stains yield results much more quickly than culturing and are especially important when infection would make an important difference in the patient's treatment and prognosis. Examples of Gram staining applications are cerebrospinal fluid for meningitis and synovial fluid for septic arthritis. The connection between disease and microorganisms has been established already in the 1800s. These studies provided important information about bacterial function in isolation. However, it became apparent that most microorganisms live in complex communities, many members of which are actually impossible to culture in isolation. Today, we know that the microorganisms or the microbiota that make up the human microbiome are not just unicellular organisms living alongside each other, but instead form highly regulated, structurally and functionally organized communities attached to surfaces as biofilms with inter-species collaborations as well as antagonisms that contribute to ecological stability. Due to the advances in metagenomic sequencing and other omics technologies, today we have enormous databases of sequence data from such communities, which has led to the emergence of computational metagenomics. This discipline allows us to study the 99% of unculturable microorganisms in the context of their natural environment.
Quorum Sensing
The stability of such communities is fascinating, as many of us probably know from trying to battle such common skin conditions as eczema, acne, and dry skin. Forming distinct communities, these microorganisms are interconnected and determined to maintain their community. Bacteria within a biofilm can communicate with each other by producing, detecting, and responding to small diffusible signal molecules in a process called quorum sensing, which confirms benefit for host colonization, biofilm formation, defense against competitors, and adaptation to changes in the environment. Quorum sensing activities and biofilms are also involved in the virulence and pathogenic potential of bacteria and are therefore an important factor in the understanding and controlling bacterial infections as they enable microorganisms and biofilms to become more tolerant of host defenses and antimicrobial agents. Inside each community, there is a balance of bacteria, fungi, and viruses that control species abundance and activity.
Human Microbiota
The human microbiota is extremely complex. It is estimated to be 10^13 to 10^14 microbial cells with around a one-to-one microbial cell to human cells ratio. These numbers are derived from the total bacterial cells in the colon, the organ that harbors the densest number of microbes. However, each one of these microorganisms carries important functional properties in genes that contain genomic information. These properties and the genomic information about microorganisms have been of growing interest to the scientific community, resulting in over 80,000 publications. The majority of the studies have focused on the intestinal microbiome. However, since the 1990s, microbiome studies were significantly advanced through such major initiatives as the Metagenomics of the Human Intestinal Tract and the subsequent Human Microbiome Project. These are huge studies that have been made possible through the advances in metagenomic sequencing. As a result, skin, lung, gut, and even brain microbiota are starting to be characterized in great detail. Each new project represents vast opportunities as well as challenges to determine which microorganisms play key roles in the development of certain environmental conditions that are needed for the healthy function of human organs, and which can be harmful to it. Over the past decade, more than 1.7 billion US dollars have been spent on human microbiome research. Major projects are also underway in the United States, the European Union, China, Canada, Ireland, South Korea, and Japan. This investment has confirmed the importance of the microbiome to human health and development. Some 20 percent of US investment in such research has gone to two phases of a huge project called the Human Microbiome Project or HMP, which is creating the research resources needed for studying the human microbiome. One of the main objectives of the HMP, launched in 2007, was to create a toolbox of reference data sets, computational techniques, analytical methods, and clinical protocols. Many of the findings from this project are what we will focus on in this program. It is because of the huge investment of capital and human research that we know that the endogenous human microbial communities contribute to critical metabolic, physiological, and immunological functions, including differentiation and maturation of the immune system, food digestion and nutrition, energy generation, metabolic regulation and control of fat storage, processing and detoxification of environmental chemicals, and maintenance of skin and mucosa barrier function, as well as development and regulation of the pro-inflammatory and anti-inflammatory processes and the prevention of invasion and growth of disease-promoting microorganisms.
Metagenomics
Metagenomics is the genomic analysis of microbial DNA from environmental communities. Metagenomics tools enable the population analysis of unculturable or previously unknown microbes. The ability to identify microbes without a priori knowledge of what sample contains is opening new doors in disciplines like microbial ecology, virology, microbiology, environmental sciences, and biomedical research.
Sequencing
Unlike other omics, metagenomics does not study a single organism but the whole community at once. It provides us with a snapshot of a collection of organisms directly in their natural environment. Since the genomes are so diverse, this is typically accomplished by sequencing a specific marker portion of the genome instead of all the genomic material present in a given sample. Such marker gene has been the 16s ribosomal RNA. 16s amplicon sequencing is a recently developed technique that is widely used to study microbiome directly in its environment. This metagenomic sequencing focuses on the 16s or the 18s gene within the small subunit of the ribosomal RNA. It has to be amplified with PCR amplification, and that's why it's called amplicon sequencing. The 16s gene sequences contain hypervariable regions, also known as HVRs, that provide species-specific signature sequences used for bacterial identification. The benefit of amplification is tied to the nature of sequencing read length, which can maximize effective output from affordable sequencing technologies. To account for the diversity of such sequences, they are usually combined into operational taxonomic units or OTUs, which can be used to classify groups of closely related individual sequences. OTUs are formed from groups of closely related genomic sequences and represent a cluster or a phylogenetic clade that has a meaningful abundance in a sample.
OTUs
Such OTU sequences are annotated by mapping to a reference database such as the Ribosomal Database Project or RDP, Green Genes, or the Silva database. Such databases contain taxonomic information for the domains of bacteria, archaea, and eukarya based primarily on phylogenies for small subunit ribosomal RNAs. Based on the mapping of specific regions amplified from the 16s ribosomal RNA gene and the small subunit of the bacterial ribosome, OTUs can be assigned to belong to a taxonomic class of bacteria, yielding a proportionate view of the community. Each community can then be studied by comparing with other communities or between individuals and conditions. Such analysis requires an in-depth understanding of data preparation methods, reference databases, as well as analytical approaches to answer specific questions based on solid evidence in the data. Importantly, sequencing data represents only one type of microbial omics, with many more becoming increasingly available. In fact, many questions about the role of microbiome members in communities are now being answered using next-generation sequencing as well as mass spectroscopy approaches for proteomics and metabolomics. Indeed, it seems like the next phase of microbiome research lies in the ability to integrate multi-omics data sets that combine next-generation sequencing, mass spec, and structural data with detailed phenotypic information about overall changes and the results of changes in the community and the environment it produces.
Resources
Now you have learned about the history of microbiology, the emergence of sequencing technologies, and how such data can be used to study microbial samples. So let's now discuss additional resources that you can rely on in this program to study these topics in greater detail. These will also include practical analysis tools for you to get experience with such data sets. In the following sessions, we will look at example data sets and run analysis together. But for now, let me give you a brief overview of the tools, methods, and project examples associated with this program. The materials in this program are expanded in our metagenomics core series, which includes Introduction to Metagenomics, Metagenomics 1, 2, and 3. Each module in these courses is designed to provide an explanation on rationale and objective, provide engaging activities, additional multimedia resources, and tutorials with examples and practical applications. Importantly, the course material will be reviewed in module quizzes and tests. These are timed and tied with overall student progress. The modules also explain key terminology as well as explain some of the project data sets that we will use in the analysis. Let's now talk a little bit about the practical analysis approaches that we will cover in this program. Throughout the course, you will be given directions to access the T-BioInfo platform for bioinformatics processing and analysis of metagenomic data. The platform includes demo pipelines as well as data management and analysis cloud infrastructure to run bioinformatics pipelines on large data sets. Different stages of analysis are performed in different sections of this multi-omics platform. The visual interface of the T-BioInfo platform will enable users to quickly build logical graphs of their analysis and understand each step's significance and role in what's called a bioinformatics pipeline. For those interested in implementing the pipeline on their own, we will offer guidance and references that can be used to effectively install all of the needed packages that are explained in the program. Behind many of the methods are scripts written in popular coding languages such as R and Python. These are also explained in tutorials that you will have access to. These are beginner tutorials on data importing, preparation for analysis, and standard visualization of processing data, as well as more specialized packages with publication-ready outputs that will also be covered in more detailed sessions.
Applications
So what kind of projects can be studied using metagenomic sequencing, and how will we get data for these projects? There are many applications of microbial research that include skin conditions, food sensitivity of the gut, autoimmune disorders, cancer, antibiotic resistance, drug resistance, and even ecological studies. In this program, we will learn to find and analyze data from such repositories as the Human Microbiome Project, which includes a data set called the American Gut. This is a citizen science project that has a lot to offer for those interested in basic microbial composition of different types of samples from healthy and mildly sick or unhealthy individuals. But we will also look at more controlled studies where animal models were used to separate the roles of environment, lifestyle, and genetic inheritance to study microbiome association with such conditions as anxiety following the gut-brain axis. Other examples we will review include studies of microbial drug resistance, viral adaptation, and analysis of soil bacteria. But microbiome seems to be involved in many more important areas of biomedical research, for example, screening for diagnostic signatures in precision medicine and association with treatment efficacy, or the therapeutic considerations for microbiome transplantation study such conditions as Alzheimer's disease. That's why we are not just going to focus on the examples that we provide, but we will provide you with a framework on how to go from data to integration and analysis that leads you to ask specific questions and rely on data to be able to perform the analysis independently. After completing this program, you should be able to find, analyze, and interpret metagenomic data on your own. If you have any questions along the way, you can always post a question on the program forum. Before the next session, we ask you to start working through the metagenomics course series below this video. You will also find important links to additional reading material on metagenomics and associated bioinformatics methods.
以上です。お役に立てれば幸いです。