レビュー論文:カンナビジオール (CBD) の経口毒性のレビュー(Review of the oral toxicity of cannabidiol (CBD))
Author:
Jeremy Gingrich, Supratim Choudhuri, Patrick Cournoyer, Jason Downey, Kristi Muldoon Jacobs
Publication: Food and Chemical Toxicology
Publisher: Elsevier
Date: June 2023
出典:https://www.sciencedirect.com/science/article/pii/S0278691523002016
https://doi.org/10.1016/j.fct.2023.113799
Under a Creative Commons license
これは、 Creative Commons Attribution License (CC BY)の条件に基づいて配布されるオープンアクセス記事です。元の著者と著作権所有者をクレジットし、このジャーナルの元の出版物を引用することを条件として、他のフォーラムでの使用、配布、または複製が許可されます。これらの条件に従わない使用、配布、または複製は許可されません。
Highlights
・Potential hazards from long term oral use of CBD are discussed.
・CBD-induced male reproductive toxicity is observed from invertebrates to primates.
・Mechanisms of CBD-mediated oral toxicity are not fully understood.
・CBDの長期経口摂取による潜在的危険性について議論した。
・CBD誘発性の雄生殖毒性は無脊椎動物から霊長類まで観察される。
・CBDを介した経口毒性の機序は完全には解明されていない。
Abstract
Information in the published literature indicates that consumption of CBD can result in developmental and reproductive toxicity and hepatotoxicity outcomes in animal models. The trend of CBD-induced male reproductive toxicity has been observed in phylogenetically disparate organisms, from invertebrates to non-human primates. CBD has also been shown to inhibit various cytochrome P450 enzymes and certain efflux transporters, resulting in the potential for drug-drug interactions and cellular accumulation of xenobiotics that are normally transported out of the cell. The mechanisms of CBD-mediated toxicity are not fully understood, but they may involve disruption of critical metabolic pathways and liver enzyme functions, receptor-specific binding activity, disruption of testosterone steroidogenesis, inhibition of reuptake and degradation of endocannabinoids, and the triggering of oxidative stress. The toxicological profile of CBD raises safety concerns, especially for long term consumption by the general population.
公表された文献の情報は、CBDの摂取が動物モデルにおいて発生および生殖毒性と肝毒性の結果をもたらす可能性があることを示している。CBD誘発性の雄生殖毒性の傾向は、無脊椎動物から非ヒト霊長類まで、系統発生的に異なる生物で観察されている。CBDはまた、種々のシトクロムP450酵素および特定の排出輸送体を阻害することが示されており、その結果、薬物‐薬物相互作用および通常は細胞外に輸送される生体異物の細胞内蓄積の可能性が生じる。CBDを介した毒性の機序は完全には解明されていないが、重要な代謝経路および肝酵素機能の破壊、受容体特異的結合活性、テストステロンステロイド産生の破壊、内因性カンナビノイドの再取り込みおよび分解の阻害、酸化ストレスの誘発が関与している可能性がある。CBDの毒性プロファイルは、特に一般集団による長期消費に対して安全性の懸念を提起する。
1. Introduction and background
Cannabidiol (CBD) and delta-9-tetrahydrocannabinol (THC) are the predominant bioactive compounds produced by cannabis (Cannabis sativa L.), an annual, herbaceous flowering plant. Cannabis has been used in many parts of the world for centuries for its fiber, edible seeds, and bioactive properties (Ren et al., 2021).
カンナビジオール(CBD)とデルタ‐9‐テトラヒドロカンナビノール (THC) は、1年生の草本性顕花植物である大麻(Cannabis sativa L.)によって生産される主要な生物活性化合物である。大麻は、繊維、食用種子、および生物活性特性のために、何世紀にもわたって世界の多くの地域で使用されてきた(Ren et al., 2021)。
Cannabis varieties have been classified into two broad categories based on their THC content, the substance predominantly responsible for the intoxicating psychological effects associated with the plant (Aizpurua-Olaizola et al., 2016). Cannabis varieties with high THC content, sometimes referred to as “marijuana”, have in many cases undergone selective breeding to increase levels of THC, thereby increasing their intoxicating effects (Gulck and Moller, 2020). Other cannabis varieties, known as hemp or industrial hemp, have relatively low THC content and have historically been cultivated for fiber and seed. The 2018 Agriculture Improvement Act defined hemp as the plant Cannabis sativa L. and any part of that plant with a THC concentration of no more than 0.3 percent on a dry weight basis (Congress, 2017–2018). Recently, access to hemp and hemp derivatives, including CBD, has increased in the United States, as the 2018 Agriculture Improvement Act removed hemp from schedule 1 of the Controlled Substances Act (USDA, 2019).
大麻の種類は、THCの含有量に基づいて2個の大きなカテゴリーに分類されている。THCは、主に植物に関連する精神的な中毒作用の原因となる物質である(Aizpurua-Olaizola et al., 2016)。THC含有量の高い大麻品種は、時に「マリファナ」と呼ばれ、多くの場合、THCのレベルを増加させるために選択的品種改良が行われ、それによって中毒効果が増加している(Gulck and Moller, 2020)。ヘンプまたは産業用ヘンプとして知られる他の大麻品種は、THC含有量が比較的低く、歴史的に繊維および種子のために栽培されてきた。2018年の農業改善法は、ヘンプを植物Cannabis sativa L.およびその植物のTHC濃度が乾燥重量ベースで0.3%以下の部分と定義した(Congress, 2017–2018)。2018年の農業改善法が規制物質法(合衆国農務省, 2019)のスケジュール1から大麻を削除したため、最近、米国ではCBDを含む大麻および大麻誘導体へのアクセスが増加している。
An increasing number of studies have investigated CBD's reported anti-inflammatory and neuropharmacological properties, including antiepileptic, sedative, anxiolytic, and antipsychotic activities (Cunha et al., 1980; Zuardi et al., 2006). These studies have raised the possibility of CBD's use in treating diverse clinical conditions like dementia, cerebral ischemia, diabetes, inflammatory diseases, nausea, and psychiatric disorders (van den Elsen et al., 2014; Wallace et al., 2015; Hayakawa et al., 2010). CBD is the active ingredient in the drug Epidiolex, which the United States Food and Drug Administration (FDA) approved for the treatment of seizures associated with Lennox-Gastaut syndrome and Dravet syndrome in 2018 and with tuberous sclerosis complex in 2020. FDA's approval of this drug is specific to the context of these severe medical conditions and patients are regularly monitored for potential adverse effects.
抗てんかん、鎮静、抗不安、および抗精神病活性を含むCBDの報告された抗炎症および神経薬理学的特性を調査する研究が増えている(Cunha et al., 1980;Zuardi et al., 2006)。これらの研究は、認知症、脳虚血、糖尿病、炎症性疾患、悪心および精神疾患のような多様な臨床状態の治療におけるCBD使用の可能性を提起した(van den Elsen et al., 2014;Wallace et al., 2015;Hayakawa et al., 2010)。CBDはEpidiolexという薬剤の有効成分であり、米国食品医薬品局 (FDA) は、2018年にLennox-Gastaut症候群およびDravet症候群に関連する発作の治療薬として、2020年に結節性硬化症に関連する発作の治療薬として承認している。FDAによる本剤の承認は、これらの重篤な医学的状態に特有のものであり、患者は潜在的な有害作用について定期的に監視される。
CBD consumer products, including CBD-containing foods, are now being marketed in many countries for their purported beneficial or general wellness effects. Top self-reported reasons for using CBD include pain, insomnia, and anxiety (Ou et al., 2020). However, toxicity studies indicate that orally consumed CBD can cause significant toxicological effects, including adverse effects on the male reproductive system and liver.
CBD含有食品を含むCBD消費製品は、現在、多くの国で、その有益なまたは一般的な健康効果のために市販されている。自己申告によるCBD使用の理由の上位は、疼痛、不眠症、不安などである(Ou et al., 2020)。しかしながら、毒性研究は、経口摂取されたCBDが、雄の生殖系および肝臓への有害作用を含む重大な毒性学的影響を引き起こす可能性があることを示している。
Substances added to food are held to a high safety standard. They are expected to be safe for all consumers over their lifetime. Therefore, evaluation of safety requires considering long-term use and use within various segments of the population, including vulnerable subpopulations such as pregnant women, the conceptus, the fetus, young children, and the elderly. Foods used as a vehicle to administer pharmacologically active substances are prone to unintentional consumption by both adults and children.
食品に添加される物質は高い安全基準に課せられている。それらは生涯にわたってすべての消費者にとって安全であることが期待される。したがって、安全性の評価には、長期使用および妊婦、受胎産物、胎児、幼児および高齢者などの脆弱な部分集団を含む様々な集団セグメントにおける使用を考慮する必要がある。薬理活性物質を投与するための媒体として使用される食品は、成人および小児の両方が意図せずに摂取する傾向がある。
Unlike FDA-approved drugs, risks from a substance added to food are not weighed against benefits demonstrated by adequate clinical trials. The drug regulatory pathway allows for risk management options not in the food ingredient regulatory framework. For example, the drug pathway entails authority to mandate labeling with detailed instructions and warnings, precise dose control, prescription and behind counter dispensation, and a Risk Evaluation and Mitigation Strategy program.
FDA承認薬とは異なり、食品に添加された物質によるリスクは、適切な臨床試験で示された便益と比較検討されていない。医薬品規制経路は、食品成分規制の枠組みにはないリスク管理の選択肢を可能にする。例えば、薬剤の経路には、詳細な指示および警告の表示、正確な用量管理、処方薬および処方箋なしで購入できる調剤、ならびにリスク評価および軽減戦略プログラムを義務付ける権限がある。
CBD is sometimes added to consumer products in the form of CBD-rich hemp extracts, which contain other plant-derived constituents. The composition of these extracts can vary considerably, depending on the source material and method of manufacturing. The other components in hemp extracts may raise additional safety questions and may interact with CBD toxicologically (Pennypacker and Romero-Sandoval, 2020). This review focuses on studies using relatively pure CBD.
CBDは、他の植物由来成分を含むCBD豊富な大麻抽出物の形で消費者製品に添加されることがある。これらの抽出物の組成は、原料および製造方法に依存してかなり変化し得る。ヘンプの抽出物中の他の成分は、さらなる安全性の疑問を提起し、CBDと毒性学的に相互作用する可能性がある(Pennypacker and Romero-Sandoval, 2020)。本レビューは、比較的純粋なCBDを用いた研究に焦点を当てる。
This review summarizes data and information in the scientific literature pertinent to the evaluation of various toxicological concerns associated with oral CBD consumption. Emphasis is placed on in vivo studies using orally administered CBD and on in vitro studies examining specific cellular effects of CBD. This review is not a risk assessment and does not seek to identify levels of exposure that may result in adverse effects or levels of exposure that are safe for test animals or for humans. This review is intended to enable thorough toxicological hazard identification for orally consumed CBD and to aid in future risk assessments.
本レビューは、経口CBD消費に関連する様々な毒性学的懸念の評価に関連する科学文献のデータと情報を要約する。経口投与されたCBDを用いたin vivo研究とCBDの特異的細胞効果を調べるin vitro研究に重点を置いた。このレビューはリスク評価ではなく、有害作用を引き起こす可能性のある暴露レベルや、試験動物やヒトにとって安全な暴露レベルを特定しようとするものではない。このレビューは、経口摂取されたCBDの徹底的な毒性学的危険性の特定を可能にし、将来のリスク評価を支援することを意図している。
1.1. The endocannabinoid system(内因性カンナビノイドシステム)
The receptors that bind to and mediate the physiological effects of THC were first discovered in the 1990s (Sugiura and Waku, 2002). This led to the discovery of the cannabinoid receptors’ endogenous ligands. These molecules are referred to as endocannabinoids to distinguish them from plant-derived cannabinoids, or phytocannabinoids. The endocannabinoid system is now known to exist in nearly all animal phyla and consists of two known cannabinoid receptors, cannabinoid receptors 1 and 2 (CB1 and CB2), and two known endocannabinoids, N-arachidonoylethanolamine (anandamide, AEA) and 2-arachidonoylglycerol (2-AG) (Silver, 2019). Both AEA and 2-AG have moderate, non-selective binding affinities for CB1 and CB2 (Soethoudt et al., 2017). Since its discovery, the endocannabinoid system has been implicated in many physiological processes in several organ systems. These processes and systems, which could be disrupted by CBD consumption, include neuronal and immune cell signaling and the reproductive systems of both sexes.
THCに結合し、THCの生理的作用を媒介する受容体は、1990年代に初めて発見された(Sugiura and Waku, 2002)。これがカンナビノイド受容体の内因性リガンドの発見につながった。これらの分子は、植物由来のカンナビノイドまたはフィトカンナビノイドと区別するために、内因性カンナビノイドと呼ばれる。内在性カンナビノイド系は現在、ほぼ全ての動物門に存在することが知られており、2個の既知カンナビノイド受容体、カンナビノイド受容体1および2 (CB1およびCB2)、ならびに2個の既知内在性カンナビノイド、N‐アラキドノイルエタノールアミン(アナンダミド、AEA)および2‐アラキドノイルグリセロール (2‐AG)から成る (Silver, 2019)。AEAおよび2-AGはいずれも、CB1およびCB2に対して中程度の非選択的結合親和性を有する (Soethoudt et al., 2017)。その発見以来、内在性カンナビノイド系はいくつかの器官系における多くの生理学的過程に関与している。CBDの消費によって破壊される可能性のあるこれらのプロセスとシステムには、両性の神経細胞と免疫細胞のシグナル伝達と生殖システムが含まれる。
The first identified cannabinoid receptor, CB1, was cloned from the rat brain, structurally classified as a seven-transmembrane G-protein coupled receptor (GPCR), and shown to be THC-responsive (Matsuda et al., 1990). The human and rat cannabinoid receptor nucleotide sequences are 90% identical and the amino acid sequences are 98% identical (Gerard et al., 1990), suggesting CB1 has a conserved physiological function across species. CB1 is expressed throughout the body, but at the highest levels in brain, endocrine, respiratory, connective, and male and female reproductive tissues (Uhlen et al., 2015). The central nervous system's response to THC is believed to be mediated exclusively by CB1, which is found locally at the axon terminals of central and peripheral neurons (Munro et al., 1993). Activation of CB1 can inhibit the release of several different excitatory and inhibitory neurotransmitters.
最初に同定されたカンナビノイド受容体CB1は、ラット脳からクローン化され、構造的に7回膜貫通G蛋白質共役受容体 (GPCR) として分類され、THC応答性であることが示された(Matsuda et al., 1990)。ヒトとラットのカンナビノイド受容体ヌクレオチド配列は90%同一であり、アミノ酸配列は98%同一であり(Gerard et al., 1990)、CB1が種を越えて保存された生理学的機能を有することを示唆する。CB1は全身に発現しているが、脳、内分泌、呼吸器、結合組織、および男性と女性の生殖組織で最高レベルで発現している(Uhlen et al., 2015)。THCに対する中枢神経系の反応は、中枢および末梢ニューロンの軸索末端に局在するCB1によってのみ媒介されると考えられている(Munro et al., 1993)。CB1の活性化は、いくつかの異なる興奮性および抑制性神経伝達物質の放出を阻害することができる。
CB2 was cloned a few years later, and its amino acid sequence is 44% identical to that of CB1 (Munro et al., 1993). Like CB1, CB2 is also expressed throughout the body, but has a different tissue-expression pattern, where the highest levels occur in endocrine, gastrointestinal, kidney, female reproductive, skin, and lymphoid tissues (Uhlen et al., 2015). Differences in amino acid sequence and in tissue expression patterns between the two receptors may reflect a functional specialization, with CB1 having been shown to regulate neurotransmitter signaling, and CB2 having implications in immune and inflammatory signaling (Pertwee et al., 2010).
CB2は数年後にクローニングされ、そのアミノ酸配列はCB1 (Munro et al., 1993)と44%同一であった。CB1と同様に、CB2も全身で発現しているが、異なる組織発現パターンを有し、最高レベルは内分泌、消化管、腎臓、女性生殖器、皮膚、およびリンパ組織で生じる(Uhlen et al., 2015)。2個の受容体間のアミノ酸配列および組織発現パターンの違いは機能的特殊化を反映している可能性があり、CB1は神経伝達物質シグナル伝達を調節することが示されており、CB2は免疫および炎症シグナル伝達に関与している(Pertwee et al., 2010)。
Endocannabinoids are produced and released by cells in response to an external stimulus (e.g., pain), act locally, and are inactivated by cellular reuptake and intracellular hydrolysis. The two enzymes responsible for hydrolyzing and inactivating AEA and 2-AG, are fatty acid amide hydrolase (FAAH) and monoacyl glycerol lipase (MAGL), respectively (Barrie and Manolios, 2017). Fatty acid binding proteins (FABPs) can act as intracellular carriers that present AEA to FAAH for reuptake and turnover (Deutsch, 2016). CBD was reported to indirectly inhibit reuptake and catabolism of AEA by binding to FABPs, thereby inhibiting their activity in AEA turnover (Fig. 1). CBD-mediated inhibition of AEA and 2-AG hydrolysis, reuptake, and turnover can prolong the antinociceptive and anti-inflammatory effects of both endocannabinoids (Barrie and Manolios, 2017).
内因性カンナビノイドは、外部刺激(例えば、痛み)に応答して細胞によって産生され放出され、局所的に作用し、細胞の再取り込みおよび細胞内加水分解によって不活性化される。AEAおよび2-AGの加水分解および不活性化に関与する2個の酵素は、それぞれ脂肪酸アミドヒドロラーゼ (FAAH) およびモノアシルグリセロールリパーゼ (MAGL) である(Barrie and Manolios, 2017)。脂肪酸結合蛋白質 (FABP) は、再取り込みと代謝回転のためにFAAHにAEAを提示する細胞内キャリアとして作用することができる (Deutsch, 2016)。CBDは、FABPに結合することによってAEAの再取り込みと異化を間接的に阻害し、それによってAEAの代謝回転におけるそれらの活性を阻害することが報告されている (図1)。AEAおよび2‐AGの加水分解、再取り込み、および代謝回転のCBD媒介阻害は、両エンドカンナビノイドの抗侵害作用および抗炎症作用を延長することができる (Barrie and Manolios, 2017)。
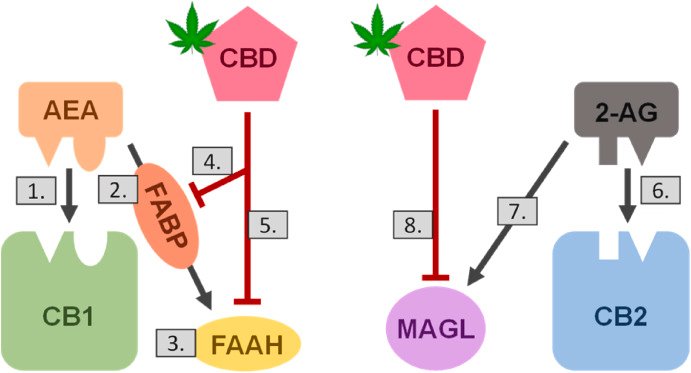
Fig. 1. CBD disrupts physiological endocannabinoid signaling. The endocannabinoids anandamide (AEA) and 2-arachidonoylglycerol (2-AG) are released locally by cells in response to an external stimulus and can act through two known pathways. Under normal conditions, AEA binds to the cannabinoid receptor 1 (CB1) to elicit a cellular response (1.) and is then presented via fatty acid binding proteins (FABP; 2.) to fatty acid amide hydrolase (FAAH) for hydrolysis (3.). CBD has been shown to inhibit both FABP presentation (4.) and FAAH hydrolysis (5.) of AEA. 2-AG, which has a stronger affinity for CB2 than CB1, first binds to CB2 to elicit a cellular response (6.) and is then inactivated by monoacyl glycerol lipase (MAGL; 7.). CBD has been shown to inhibit MAGL activity (8.). These disruptions of CBD to the endocannabinoid system could result in prolonged endocannabinoid signaling due to decreased hydrolysis, reuptake, and turnover of AEA and 2-AG.
図1。CBD は生理的な内因性カンナビノイドシグナル伝達を妨害します。
内在性カンナビノイドであるアナンダミド (AEA) と2‐アラキドノイルグリセロール (2‐AG) は、外部刺激に応答して細胞から局所的に放出され、2個の既知経路を介して作用することができる。正常条件下では、AEAはカンナビノイド受容体1 (CB1) に結合して細胞応答を誘発し (1.)、脂肪酸結合蛋白質(FABP;2.)を介して脂肪酸アミドヒドロラーゼ (FAAH) に提示されて加水分解される(3.)。CBDは、AEAのFABP提示 (4.) およびFAAH加水分解 (5.) の両方を阻害することが示されている。CB1よりもCB2に対してより強い親和性を有する2-AGは、まずCB2に結合して細胞応答 (6.) を誘発し、次いでモノアシルグリセロールリパーゼ(MAGL; 7.)によって不活性化される。CBDはMAGL活性を阻害することが示されている (8)。内因性カンナビノイドシステムに対するCBDのこれらの破壊は、AEAおよび2‐AGの加水分解、再取り込み、および代謝回転の低下による内因性カンナビノイドシグナル伝達の延長をもたらす可能性がある。
1.2. Receptor binding profile of CBD(CBDの受容体結合プロファイル)
Although there is a high structural similarity between THC and CBD, CBD has a weak binding affinity for either CB1 or CB2 (McPartland et al., 2015; Shahbazi et al., 2020), and to date, there is no substantive evidence that CBD causes THC-like psychotropic effects (WHO, 2018). Thus, CBD's mechanism of action must occur, in part, through targets other than canonical cannabinoid receptor binding. Despite having weak affinity for CB1 and CB2, CBD can act as a non-competitive negative allosteric modulator for CB1 in the presence of THC and is able to reduce the efficacy and potency of both THC and the endocannabinoid AEA (Laprairie et al., 2015; Thomas et al., 2007).
THCとCBDの間には高い構造的類似性があるが、CBDはCB1またはCB2のいずれかに対して弱い結合親和性を有し(McPartland et al., 2015;Shahbazi et al., 2020)、現在まで、CBDがTHC様向精神作用を引き起こすという実質的な証拠はない(WHO, 2018)。したがって、CBDの作用機序の一部は、標準的なカンナビノイド受容体結合以外の標的を介して起こるに違いない。CB1およびCB2に対して弱い親和性を有するにもかかわらず、CBDはTHCの存在下でCB1に対する非競合的ネガティブアロステリック調節因子として作用することができ、THCおよび内因性カンナビノイドAEAの両方の有効性および効力を低下させることができる (Laprairie et al., 2015;Thomas et al., 2007)。
CBD has been reported to activate the vanilloid type 1 receptor (TRPV1), a non-specific cation channel involved in nociceptive signaling (Bisogno et al., 2001). When activated by CBD (EC50 = 3.2–3.5 μM), TRPV1 causes an increase in intracellular Ca2+. In vitro, this can cause desensitization of TRPV1 to its natural ligand, capsaicin, suggesting that TRPV1 receptors could mediate some of the toxicological effects of CBD (Bisogno et al., 2001).
CBDは、侵害シグナル伝達に関与する非特異的陽イオンチャネルであるバニロイド1型受容体 (TRPV1) を活性化することが報告されている (Bisogno et al., 2001)。CBDによって活性化されると (EC50=3.2–3.5μM)、TRPV1は細胞内Ca2+の増加を引き起こす。in vitroでは、これは天然リガンドであるカプサイシンに対するTRPV1の脱感作を引き起こす可能性があり、TRPV1受容体がCBDの毒性作用の一部を媒介できることを示唆している(Bisogno et al., 2001)。
Recently, CBD was demonstrated to interact with the D1-like dopamine receptors in the hippocampus (Nouri et al., 2021), which are known to play a crucial role in regulating the reward properties of methamphetamine and other drugs. The authors reported that CBD's interaction with D1-like dopamine receptors inhibited the reward effect of methamphetamine in rats.
最近、CBDは海馬のD1様ドーパミン受容体と相互作用することが示され (Nouri et al., 2021)、それはメタンフェタミンや他の薬物の報酬特性の調節に重要な役割を果たすことが知られている。著者らは、CBDとD1様ドーパミン受容体との相互作用が、ラットにおけるメタンフェタミンの報酬効果を阻害することを報告した。
Studies suggest additional receptors could be involved in CBD's mechanisms of action, such as G-protein coupled receptor 55 (GPR55), serotonin receptor subtype 1A (5-HT1A), and dopamine receptor (D2) (Galaj and Xi, 2020). In rats, CBD reduced neuronal hyperexcitability and inflammation through modulation of adenosine-mediated signaling and intracellular calcium levels via interaction with GPR55 and TRPV1 (FDA, 2018a). In mice, CBD exerted an analgesic effect through positive allosteric modulation of α3 glycine receptors by directly binding to the receptor; this effect was not observed in α3 glycine receptors-null mice (Xiong et al., 2011). Other studies suggest CBD interacts with many other receptors, such as PPAR-γ, α1-adrenoceptors, GABAA, and μ- and δ-opioid receptors, with varying efficacy (McPartland et al., 2015).
研究は、G蛋白質共役受容体55 (GPR)、セロトニン受容体サブタイプ1A (5‐HT1A) およびドーパミン受容体 (D2)など、追加の受容体がCBDの作用機序に関与する可能性を示唆している(Galaj and Xi, 2020)。ラットにおいて、CBDは、GPR55およびTRPV1との相互作用を介したアデノシン媒介シグナル伝達および細胞内カルシウムレベルの調節を介して、ニューロンの過剰興奮性および炎症を低下させた(FDA, 2018a)。マウスでは、CBDはα3グリシン受容体に直接結合することにより、α3グリシン受容体の正のアロステリック調節を介して鎮痛作用を発揮した;この作用はα3グリシン受容体欠損マウスでは認められなかった (Xiong et al., 2011)。その他の研究では、CBDはPPAR-γ、α1アドレナリン受容体、GABAA、μおよびδオピオイド受容体など、他の多くの受容体と相互作用し、その効力は様々であることが示唆されている (McPartland et al., 2015)。
Together, these data suggest CBD has a non-selective receptor binding profile. Since the mechanisms by which CBD elicits its toxicological effects are still poorly understood, it remains unclear exactly which receptors are implicated for which endpoints, but it is likely multifarious.
まとめると、これらのデータは、CBDが非選択的な受容体結合プロファイルを有することを示唆している。CBDがその毒性作用を誘発するメカニズムはまだほとんど理解されていないため、どの受容体がどのエンドポイントに関与しているかは正確には不明であるが、おそらく多様である。
It is important to note that CBD occurs as both a (+) and (−) enantiomer. The (−) enantiomer is the plant-derived form of CBD, the primary subject of study in the literature, and the active ingredient in the drug Epidiolex. Chemical synthesis of CBD can result in either the (+) and (−) enantiomer, depending on the starting material. Interestingly, CBD's (+) enantiomer demonstrates a different receptor binding profile than described above. The Ki of the CBD (+) enantiomer for CB1 and CB2 receptors is much lower than the (−) enantiomer, suggesting that the (+) enantiomer has a far greater binding affinity for the cannabinoid receptors (Morales et al., 2017). The CBD (+) enantiomer was also found to be more active than the CBD (−) enantiomer as an anticonvulsant agent in a mouse seizure model (Leite et al., 1982). These noted pharmacological differences between (+) and (−) CBD enantiomers will be important to consider should synthetically derived CBD become prevalent.
CBDは (+) および (−) 鏡像異性体として存在することに注意することが重要である。(−) 鏡像異性体は植物由来のCBDであり、文献における主要な研究対象であり、薬剤Epidiolexの有効成分である。CBDの化学合成は、出発物質に依存して (+) または (−) 鏡像異性体のいずれかを生じる。興味深いことに、CBDの (+) 鏡像異性体は、上記とは異なる受容体結合プロファイルを示す。CBD (+) 鏡像異性体のCB1およびCB2受容体に対するKiは (−) 鏡像異性体よりはるかに低く、(+)鏡像異性体がカンナビノイド受容体に対してはるかに高い結合親和性を有することを示唆している(Morales et al., 2017)。また、マウス痙攣モデルにおいて、CBD (+)鏡像異性体は、CBD (−)鏡像異性体よりも抗痙攣薬としてより活性であることが見出された(Leite et al., 1982)。(+) および (−) CBD鏡像異性体間のこれらの注目された薬理学的相違は、合成由来CBDが普及する場合に考慮することが重要である。
1.3. Toxicokinetics of CBD(CBDの毒物動態)
Millar et al. (2018) conducted a systematic review of published articles retrieved from PubMed and EMBASE that reported toxicokinetic (TK) data on CBD in humans. The mean half-life (t1/2) of CBD was reported to be 1.44–10.86 h after oromucosal spray (5–20 mg), 1.09–1.97 h after single oral administration (10 or 20 mg), 2–5 days after chronic oral administration, 24 h after intravenous administration, and 31 h after inhalation. The area-under-the-curve (AUC) and maximum serum concentration achieved (Cmax) increased in a dose-dependent manner and were higher following inhalation relative to oromucosal spray or oral administration. Administration of CBD with a meal or in a lipid formulation also increased Cmax. The time to Cmax (Tmax) was reached between 0 and 5 h and was not dose dependent. CBD was rapidly distributed into tissues, and appears to distribute to adipose tissue due to its high lipophilicity (WHO, 2018). Mean apparent volume of distribution for CBD was estimated to be 2520 L following intravenous administration, and around 30,000 L following oromucosal spray (Millar et al., 2018).
Millar et al. (2018)は、ヒトにおけるCBDの毒物動態(TK)データを報告したPubMedおよびEMBASEから検索された公表論文の系統的レビューを実施した。CBDの平均半減期 (t1/2) は、口腔粘膜噴霧 (5~20 mg) で1.44~10.86時間、単回経口投与 (10または20 mg) で1.09~1.97時間、長期経口投与で2~5日、静脈内投与で24時間、吸入で31時間と報告されている。曲線下面積 (AUC) および達成された最大血清中濃度 (Cmax) は用量依存的に増加し、口腔粘膜スプレーまたは経口投与と比較して吸入後に高かった。食事または脂質製剤中のCBD投与もCmaxを増加させた。Cmax到達時間 (Tmax) は0~5時間であり、用量依存性はなかった。CBDは迅速に組織に分布し、その高い親油性により脂肪組織に分布するようである(WHO, 2018)。CBDの平均見かけの分布容積は、静脈内投与では2520L、口腔粘膜スプレーでは約30000Lと推定された(Millar et al., 2018)。
Some TK parameters of CBD in humans were identified in studies on pharmaceutical grade CBD as the active ingredient in the drug Epidiolex. In these studies, oral exposure to CBD resulted in a nonlinear increase in plasma CBD concentration with a dose of up to 6000 mg under fasting conditions, with the median Tmax being 2.5–5 h. Consuming CBD with a high-fat meal increased the Cmax approximately 5-fold and increased the AUC approximately 4-fold. The estimated Vd in healthy volunteers ranged from 20,963–42,849 L, similar to the oromucosal spray administration. The average elimination t1/2 was 56–61 h, and CBD was shown to be primarily excreted in feces (84%), with a small fraction (8%) excreted in urine (FDA, 2018a; FDA, 2018b). In rats, the t1/2 of CBD was within the same range as humans (4–5 h) after a single oral dose (FDA, 2018b).
ヒトにおけるCBDのいくつかのTKパラメータは、医薬品Epidiolexの活性成分として医薬品グレードのCBDに関する研究で同定された。これらの研究では、CBDの経口暴露は、絶食下で最高6000 mgまでの用量で血漿中CBD濃度の非線形増加をもたらし、Tmaxの中央値は2.5–5時間であった。高脂肪食とともにCBDを摂取すると、Cmaxは約5倍に上昇し、AUCは約4倍に上昇した。健常ボランティアにおける推定Vdは20,963–42,849 Lであり、口腔粘膜スプレー投与と同様であった。平均排泄 t1/2は56–61時間であり、CBDは主に糞便中に排泄され (84%)、少量が尿中に排泄された (8%) (FDA、2018a;FDA, 2018b)。ラットでは、単回経口投与後のCBDのt1/2はヒトと同じ範囲であった(FDA, 2018b)。
The oral bioavailability of CBD in humans is low, varying between approximately 6 and 19% (Mechoulam et al., 2002; Cherniakov et al., 2017; Millar et al., 2020; Perucca and Bialer, 2020). Oral bioavailability is lowest (∼6%) under fasting conditions, and highest (∼19%) when consumed with a high fat meal (Perucca and Bialer, 2020). Low oral bioavailability of CBD is likely due to its highly lipophilic nature (logP = 6.3), which results in poor gastric solubility (Millar et al., 2020). The oral bioavailability of CBD in rats is lower than humans, reaching only 2.8% after a single dose (FDA, 2018b).
ヒトにおけるCBDの経口バイオアベイラビリティは低く、約6%と19%の間で変動する (Mechoulam et al., 2002; Cherniakov et al., 2017; Millar et al., 2020; Perucca and Bialer, 2020)。経口バイオアベイラビリティは絶食下で最も低く(~6%)、高脂肪食を摂取すると最も高く(~19%)なる (Perucca and Bialer, 2020)。CBDの経口バイオアベイラビリティの低さは、その高い親油性(logP=6.3)に起因する可能性が高く、その結果、胃溶解性が低い (Millar et al., 2020)。ラットにおけるCBDの経口バイオアベイラビリティはヒトより低く、単回投与後にわずか2.8%に達する(FDA, 2018b)。
Taken together, these data demonstrate that the toxicokinetics of CBD markedly vary depending on route of administration and food matrix in both rats and humans. Importantly, alterations in the conditions of use or formulations of CBD that enhance its oral bioavailability in consumer products or significantly impact other toxicokinetic parameters for CBD, could increase the potential for adverse toxicological outcomes.
まとめると、これらのデータはCBDの毒物動態がラットとヒトの両方で投与経路と食物マトリックスに依存して著しく異なるものになることを示す。重要なことは、消費者製品におけるCBDの経口バイオアベイラビリティを高めたり、CBDの他のトキシコキネティクスパラメータに有意な影響を与えるようなCBDの使用条件や製剤の変更は、有害な毒性学的結果の可能性を高める可能性があるということである。
1.4. Species-specific metabolism(種特異的な代謝)
In humans, CBD is metabolized in the gut and liver, where it undergoes phase I metabolism by multiple cytochrome P450 (CYP) isoforms (primarily CYP2C19 and CYP3A4) and undergoes phase II metabolism (glucuronidation) by UDP-glucuronosyltransferases (UGT; primarily UGT1A7, UGT1A9, and UGT2B7) (FDA, 2018a; Jiang et al., 2011). Human liver microsomes can metabolize CBD to produce monohydroxylated metabolites (6α–OH–, 6β–OH–,7-OH-, 1”–OH–, 2”–OH–, 3”–OH–, 4”–OH–, and 5”–OH–CBDs) of which 6α–OH–, 6β–OH–, 7-OH-, and 4”–OH–CBDs are the most abundant (Harvey and Mechoulam, 1990). Some of these hydroxylated metabolites are further oxidized into carboxylic acid derivatives (WHO, 2018). The predominant CBD metabolite in humans is 7-COOH-CBD (Harvey et al., 1991). Consistent with this, clinical studies associated with the CBD-based drug Epidiolex reported that the plasma AUC for 7-COOH-CBD was about 40-fold higher than that of the parent compound, indicating CBD undergoes rapid metabolism in humans (FDA, 2018a).
ヒトでは、CBDは腸と肝臓で代謝され、複数のシトクロムP450 (CYP) アイソフォーム (主にCYP2C19とCYP3A4) による第 l 相代謝を受け、UDP‐グルクロノシルトランスフェラーゼ(UGT;主にUGT1A7、UGT1A9、およびUGT2B7)による第 II 相代謝 (グルクロン酸化) を受ける (FDA、2018a;Jiang et al., 2011)。ヒト肝臓ミクロソームはCBDを代謝してモノヒドロキシル化代謝物(6α–OH–、6β–OH–、7-OH-、1”–OH–、2”–OH–、3”–OH–、4”–OH–、および5”–OH–CBD)を産生することができ、そのうち6α–OH–、6β–OH–、7-OH-、および4′′–OH–CBDが最も豊富である(Harvey and Mechoulam, 1990)。これらのヒドロキシル化代謝物の一部はさらに酸化されてカルボン酸誘導体となる (WHO, 2018)。ヒトにおける主要なCBD代謝物は7-COOH-CBDである (Harvey et al., 1991)。これと一致して、CBDベースの薬剤Epidiolexに関連する臨床試験では、7-COOH-CBDの血漿中AUCが親化合物の約40倍であることが報告されており、CBDがヒトにおいて急速に代謝されることを示している (FDA, 2018a)。
In vitro studies using liver microsomes from a wide variety of mammalian species, including humans, have detected more than 50 different CBD metabolites with complex profiles and significant interspecies differences (Harvey and Brown, 1991). CBD metabolites were evaluated in vivo in dogs, rats, and humans. In dogs, 7-COOH-CBD was identified only as a minor urinary metabolite, where 4”- and 5”-hydroxy and 6-oxo-CBD were dominant. In rats, C-6 or C-7 hydroxylated metabolites predominated. No intact glucuronide or glucoside metabolites were found in either dog or rat urine (Harvey et al., 1991). In contrast, in vivo human studies report that a large portion of the administered CBD was excreted as 7-COOH-CBD or as a glucuronide-conjugate (Ujvary and Hanus, 2016).
ヒトを含む様々な哺乳類の肝ミクロソームを用いたin vitro研究では、複雑なプロファイルと有意な種間差を有する50種類以上の異なるCBD代謝物が検出された (Harvey and Brown, 1991)。CBD代謝物をイヌ、ラット、ヒトでin vivo評価した。イヌでは、7-COOH-CBDは少量の尿中代謝物としてのみ同定され、4"-および5"-ヒドロキシおよび6-オキソ-CBDが優勢であった。ラットでは、C‐6またはC‐7ヒドロキシル化代謝産物が優勢であった。イヌおよびラットの尿中には、完全なグルクロン酸抱合体またはグルコシド代謝物は認められなかった (Harvey et al., 1991)。対照的に、in vivoヒト試験では、投与されたCBDの大部分が7-COOH-CBDまたはグルクロニド複合体として排泄されたことが報告されている (Ujvary and Hanus, 2016)。
These data add to the body of literature suggesting both similarities and differences in the metabolism of CBD among mammalian species. Importantly, in the nonclinical review of Epidiolex, it is noted that these studies did not adequately assess the safety of 7-COOH-CBD due to its relatively low abundance in the animal test species compared to humans, and that this gap represents a significant deficiency that should be addressed (FDA, 2018b).
これらのデータは、哺乳類種間のCBD代謝における類似性と相違性の両方を示唆する文献に追加される。重要なことは、Epidiolexの非臨床レビューにおいて、これらの研究は、7-COOH-CBDの動物試験種における存在量がヒトと比較して相対的に低いため、その安全性を十分に評価しておらず、このギャップは対処すべき重大な欠陥を表していることを指摘している(FDA, 2018b)。
1.5. Transport proteins(輸送タンパク質)
CBD is not a substrate for the major drug efflux transporters breast cancer resistance protein (BCRP) and permeability glycoprotein (P-gp) (Iffland and Grotenhermen, 2017; Feinshtein et al., 2013). These findings could suggest CBD has poor efflux from blood-tissue barriers, which raises questions as to CBD levels in various tissues such as brain, testes, or placenta. Additionally, CBD is not a substrate for the important human hepatic uptake transporters OATP1B1 and OATP1B3, the major renal uptake transporters OAT1, OAT2, OCT2, or the major renal efflux transporter MATE1 (FDA, 2018a), which may account for its low percentage of urinary elimination.
CBDは、主要な薬物排出輸送体である乳癌耐性蛋白質 (BCRP) および透過性糖蛋白質 (P‐gp)の基質ではない (Iffland and Grotenhermen, 2017;Feinshtein et al., 2013)。これらの知見は、CBDが血液組織関門からの排出が不十分であることを示唆し、脳、精巣、胎盤などの様々な組織におけるCBDレベルに関する疑問を提起する。さらに、CBDは重要なヒト肝臓取り込み輸送体OATP1B1およびOATP1B3、主要な腎臓取り込み輸送体OAT1、OAT2、OCT2、または主要な腎臓排出輸送体であるMATE1 (FDA、2018a)の基質ではなく、これが尿中排泄率の低さの原因である可能性がある。
2. Toxicological profile of CBD(CBD の毒性プロファイル)
2.1. Developmental and reproductive toxicity(発達および生殖毒性)
2.1.1. Non-human primates(非ヒト霊長類)
A 90-day (subchronic) oral toxicity study for CBD was performed in Rhesus monkeys (Rosenkrantz et al., 1981). Animals were administered 0, 30, 100, or 300 mg/kg bw/day of CBD (99% purity) by oral intubation. Significant changes in organ weights were seen in all CBD dose groups in both sexes, although a clear dose-response was not observed for all organs. Relative organ weights were 13–56% higher for liver and 16–22% higher for kidneys compared to controls. Relative heart weight was 16–22% higher in the highest dose group (300 mg/kg bw). Significantly lower gonadal weight was observed in both sexes at all doses, including for relative ovary weight (25–75% lower) and testes size (8–25%) at day 90. After a 30-day washout period, the weight of most organs returned to normal, suggesting that the changes in relative organ weights were CBD treatment-related, and partially reversible with discontinued use. However, the liver weights remained slightly elevated, and testes size remained depressed by 20–25%. No changes in organ weights were associated with any functional impairment, except for the testes. Inhibition of spermatogenesis was observed in 2 of 4, 3 of 4, and 4 of 4 monkeys given 30, 100 and 300 mg/kg bw of CBD, respectively. This change was not observed in the control animals. Functional impairment of testes was accompanied by histological changes, including smaller seminiferous tubules, lower mitotic index, fewer germ cells per tubule, and decreased number of spermatocytes, spermatids, and spermatozoa. These data strongly suggest that orally administered CBD exerts toxic effects on the male reproductive system in primates, some of which, like testes size, may be irreversible. To note, the same researchers also conducted an acute intravenous toxicity study in Rhesus monkey, which established an LD50 for CBD (approximately 212 mg/kg bw) and reported serious adverse effects on the testes, which significantly decreased in weight (∼57%) after seven days of exposure (Rosenkrantz et al., 1981).
CBDの90日間(亜慢性)経口毒性試験がアカゲザルで実施された (Rosenkrantz et al., 1981)。動物は経口挿管によりCBD (純度99%) 0。30、100または300 mg/kg 体重/日を投与された。臓器重量の有意な変化が雌雄のすべてのCBD用量群で見られたが、すべての臓器で明確な用量反応は観察されなかった。相対臓器重量は、対照群と比較して肝臓で13–56%、腎臓で16–22%高かった。最高用量群 (300 mg/kg 体重) では、相対心臓重量が16~22%高かった。生殖腺重量の有意な減少が全用量群の雌雄で観察され、90日目の相対卵巣重量 (25–75%の減少) および精巣サイズ (8–25%の減少) も同様であった。30日間の洗浄期間後、ほとんどの臓器の重量が正常に戻ったことから、相対的な臓器重量の変化はCBD投与に関連したものであり、使用中止により部分的に回復することが示唆された。しかしながら、肝臓重量はわずかに増加したままであり、精巣の大きさは20–25%減少したままであった。精巣を除き、臓器重量の変化は機能障害と関連していなかった。30、100および300 mg/kg 体重のCBDを投与したサルでは、それぞれ2/4匹、3/4匹および4/4匹に精子形成の阻害が観察された。この変化は対照動物では観察されなかった。精巣の機能障害は、精細管の小型化、有糸分裂指数の低下、精細管当たりの生殖細胞数の減少、精母細胞、精子細胞および精子数の減少などの組織学的変化を伴った。これらのデータは、経口投与されたCBDが霊長類の雄の生殖系に毒性作用を及ぼし、その一部は精巣の大きさのように不可逆的である可能性があることを強く示唆している。なお、同研究者らはアカゲザルを用いた急性静脈内投与毒性試験も実施しており、その結果、CBDのLD50値(約212 mg/kg bw)を確立し、精巣への重篤な有害作用が報告されている、それは精巣の重量において曝露7日後に有意に減少(~57%)した(Rosenkrantz et al., 1981)。
2.1.2. Rodents(げっ歯類)
In mice, a perinatal exposure study to CBD and other cannabinoids was used to evaluate their impact on testicular function and fertility (Dalterio and deRooij, 1986). In this study, one group of females received a single oral dose of 50 mg/kg BW of CBD on day 12 of gestation, and another group of females received a single oral dose of 50 mg/kg BW of CBD within 12 h of parturition to evaluate the developmental effects of in utero and lactational exposure to CBD. In a previous study, this dose did not produce overt maternal toxicity or pup mortality (Dalterio, 1980). Female mice receiving an oral dose of 50 mg CBD/kg BW on day 12 of gestation produced male offspring with a significant reduction in testicular weight. Male mice with lactational exposure to CBD had a reduced rate of successful impregnations in adulthood compared to controls and sired significantly fewer live pups. These males had about 20% fewer viable spermatids than the controls. These findings are consistent with earlier in vitro explant studies in human and animal tissue that report cannabinoids, specifically THC, can disrupt testicular function (Bloch et al., 1978). The same group also reported that male mice exposed to CBD in adulthood had altered spermatogenesis, reduced fertility, and produced litters with significantly more prenatal and postnatal mortality (Dalterio et al., 1982).
マウスでは、CBDおよび他のカンナビノイドへの周産期曝露試験を用いて、精巣機能および生殖能力への影響を評価した(Dalterio and deRooij, 1986)。この研究では、CBDへの子宮内および授乳曝露の発達影響を評価するために、雌の1群は妊娠12日目にCBDの50 mg/kg BWの単回経口投与を受け、別の群は分娩12時間以内にCBDの50 mg/kg BWの単回経口投与を受けた。以前の試験では、この用量では明らかな母体毒性や仔動物の死亡はみられなかった (Dalterio, 1980)。妊娠12日目にCBD 50 mg/kg体重を経口投与した雌マウスは、精巣重量の有意な減少を伴う雄の子孫を産生した。受胎期にCBDに曝露された雄マウスは、対照群と比較して、成体での妊娠成功率が低く、出生仔の数も有意に少なかった。これらの雄は対照より生存精子細胞が約20%少なかった。これらの知見は、カンナビノイド、特にTHCが精巣機能を破壊する可能性があることを報告したヒトおよび動物組織における初期のin vitro外植片研究と一致している(Bloch et al., 1978)。同グループはまた、成人期にCBDに暴露した雄マウスが精子形成を変化させ、生殖能力を低下させ、出生前および出生後の死亡率が有意に高い同腹の子たちを出産したことを報告した (Dalterio et al., 1982)。
Consistent with these findings on the male reproductive toxicity of CBD, three other studies by a different group also observed that CBD administered orally to male mice during the juvenile period of postnatal development caused reproductive toxicity (Carvalho et al., 2018a, 2018b, 2022). Here, 21-day old male Swiss mice were administered CBD (99.9% pure) by gavage for 34 consecutive days at doses of 0, 15, or 30 mg/kg BW/day. Sexual behavior was analyzed for 10 days using observations like first mount, number of intromissions, ejaculation latencies, and postejaculatory mount intervals. These analyses revealed that chronic administration of 15 mg/kg BW/day of CBD reduced the frequency of mounts and the number of intromissions and ejaculations. At 30 mg/kg BW/day, CBD exposure resulted in a statistically significant reduction in fertility rate, including pre- and post-implantation embryo loss, and in the total number of litters. The average percent loss of pre- and post-implantation embryos tended to be higher in the highest dose group (30 mg/kg BW), although the inter-dose differences were not statistically significant (Carvalho et al., 2018a). In a second study by the same researchers using the same dosing strategy but with a 35-day washout period (Carvalho et al., 2018b), CBD exposure did not affect body weight or the weight of reproductive tissue such as testis, epididymis, and seminal vesicle. In the 30 mg/kg BW group, there was a significant decrease in total circulating plasma testosterone compared to controls. A decrease in total circulating plasma testosterone was also observed in the 15 mg/kg BW group, but the decrease was not statistically significant. In the 30 mg/kg BW group, a statistically significant decrease in the number of Sertoli cells was observed. However, this was not accompanied by significant differences in testicular sperm counts, although the number of spermatozoa in the epididymis tail was reduced. Spermatozoa from both dose groups had head and tail abnormalities, and a large number of cytoplasmic droplets were observed in the medial region of flagella. The authors stated that the steady state blood levels of CBD for the doses used (15 and 30 mg/kg BW) would be about 80–160 ng/ml. In a third study by the same researchers using the same dosing strategy in 21-day-old male mice (Carvalho et al., 2022), marked effects in spermatogenesis and spermatozoa performance were noted at both doses. Such effects included increases in DNA damage and number of abnormal acrosomes in mature spermatozoa and decreases in spermatozoa straight-line and average pathway velocities and in enzymatic activity of superoxide dismutase and catalase — enzymes that function to protect spermatozoa from oxidative stress. Changes in testicular morphology, including a reduced seminiferous tubule epithelium height, and changes in spermatogenesis in a stage-specific manner were also noted in CBD-exposed animals. Additionally, the highest dose (30 mg CBD/kg BW/day) resulted in reductions in spermatozoa motility, curvilinear velocity, and number of mature spermatozoa with intact acrosomes. While these three studies were not formally integrated, findings following a washout period suggest CBD-induced male reproductive toxicity effects in mice are reversible with discontinued use, which differs from reports in monkey. However, the findings on spermatogenesis are similar to those observed in adult non-human primates (Rosenkrantz et al., 1981).
CBDの雄性生殖毒性に関するこれらの知見と一致して、別のグループによる他の3件の研究でも、出生後発達の幼若期にCBDを雄マウスに経口投与すると生殖毒性が生じることが観察された(Carvalho et al., 2018a, 2018b, 2022)。ここでは、21日齢雄のスイスマウスにCBD (純度99.9%) を0、15または30 mg/kg 体重/日の用量で34日間連続で強制経口投与した。性行動は、初回マウント、挿入回数、射精潜時、および射精後マウント間隔などの観察を用いて10日間分析された。これらの分析は、CBDの15 mg/kg 体重/日の長期投与がマウンティングの頻度と挿入と射精の数を減少させることを明らかにした。30 mg/kg 体重/日で、CBD曝露は、着床前および着床後の胚損失を含む受精率および同腹仔数の統計的に有意な減少をもたらした。着床前後の胚の平均損失率は最高用量群 (30 mg/kg体重) でより高くなる傾向があったが、用量間差は統計的に有意ではなかった(Carvalho et al., 2018a)。同じ研究者が同じ投与戦略を用いて35日間の洗浄期間を設定した2番目の研究(Carvalho et al., 2018b)では、CBD暴露は体重や精巣、精巣上体、精嚢などの生殖組織の重量に影響を及ぼさなかった。30 mg/kg体重群では、対照と比較して、総循環血漿テストステロンの有意な減少があった。総循環血漿テストステロンの減少も15 mg/kg BW群で観察されたが、減少は統計学的に有意ではなかった。30 mg/kg体重群では、セルトリ細胞数の統計学的に有意な減少が観察された。しかしながら、精巣上体尾部の精子数は減少したが、精巣精子数に有意差はなかった。両用量群の精子に頭部および尾部の異常が認められ、多数の細胞質小滴が鞭毛の内側領域に観察された。著者らは、使用された用量 (15および30 mg/kg体重) における定常状態の血中CBD濃度は約80–160 ng/mlであると述べた。同じ研究者が21日齢の雄マウスに同じ投与戦略を用いて行った3番目の試験(Carvalho et al., 2022)では、精子形成と精子機能に顕著な影響が両用量で認められた。このような影響には、成熟精子におけるDNA損傷および異常な先体数の増加、精子の直線速度および平均経路速度の低下、ならびにスーパーオキシドジスムターゼおよびカタラーゼの酵素活性の低下が含まれた ー 精子を酸化ストレスから保護する酵素。精細管上皮の高さの減少を含む精巣形態の変化および段階特異的な精子形成の変化もCBD暴露された動物で認められた。さらに、最高用量 (30 mg CBD/kg 体重/日) では、精子の運動性、曲線速度、および先体が完全な成熟精子の数が減少した。これら3件の試験は正式には統合されていないが、休薬期間後の所見から、マウスにおけるCBD誘発性の雄生殖毒性は使用中止により可逆的であることが示唆されており、これはサルにおける報告とは異なる。しかしながら、精子形成に関する所見は、ヒト以外の霊長類の成体で観察された所見と類似している(Rosenkrantz et al., 1981)。
In a recent study, prenatal CBD exposure (20 mg CBD/kg BW/day) in mice, which occurred via maternal oral exposure 2-weeks prior to mating and continued through gestation and lactation, resulted in increased anxiety-like behavior in F1 female offspring (Wanner et al., 2021). Additionally, differentially methylated loci in both the cerebral cortex and hippocampus brain regions were identified. Taken together, these findings suggest that developmental exposure to CBD via maternal oral exposure may be associated with undesirable behavioral outcomes in female offspring and perturbations in the brain epigenome.
最近の研究では、マウスの出生前のCBD暴露 (CBD 20 mg/kg体重/日) が、交配2週間前に母体の経口暴露から妊娠および授乳期間中継続され、F1雌仔に不安様行動の増加をもたらした(Wanner et al., 2021)。さらに、大脳皮質と海馬脳領域の両方で異なるメチル化遺伝子座を同定した。まとめると、これらの知見は、母親の経口曝露を介したCBDへの発達上の曝露が、雌の子孫における望ましくない行動転帰および脳エピゲノムの混乱と関連する可能性があることを示唆する。
In rats, three studies were reviewed by FDA to support approval of the CBD-based drug Epidiolex, including (I) an embryofetal development study, (II) a fertility and early embryonic developmental study, and (III) a pre- and postnatal developmental toxicity study (FDA, 2018b). In all three studies, CBD was administered at doses of 0, 75, 150, and 250 mg/kg BW/day via oral gavage. Summarized below are results from these studies; conclusions are reported in the Epidiolex drug label (FDA, 2018c).
ラットでは、(I) 胚胎児発生に関する試験、(II) 生殖能力および初期胚発生に関する試験、および (III) 出生前および出生後の発生毒性試験を含む3件の試験が、CBDベースの薬剤Epidiolexの承認を支持するためにFDAによって審査されました(FDA, 2018b)。全ての研究で、CBDを0、75、150、250 mg/kg 体重/日の用量で経口強制投与した。これらの研究の結果を以下に要約する;結論は Epidiolex の薬剤ラベルに記載されている (FDA, 2018c)。
(I)
Pregnant females were exposed from gestational day (GD) 6 through 17. Two females in the high-dose group (250 mg/kg BW/day) had CBD treatment-related total litter loss in utero. There were statistically significant differences compared to controls in the occurrence of fetal structural variations, like supernumerary liver lobe in the high dose group, which was associated with maternal plasma levels greater than those expected in humans.
(II)
Male and female rats were exposed from 2 weeks prior to mating through GD 6. Lower overall body weight gains were observed in mid- and high-dose males; body weight gain was not affected in females. One mid-dose male and one high-dose male failed to impregnate a female. At necropsy, there were decreases in male reproductive organ weights at all doses tested.
(III)
Pregnant females were exposed from GD 6 through pregnancy and lactation (to postnatal day [PND] 21). Here, fur staining in the urogenital area was observed at all doses; increased incidences of pups with attached umbilical cords, small pup size, shorter gestation, noisy respiration, delayed achievement of developmental landmarks (i.e., pinna unfolding, eye opening, and pupillary reflex), delayed sexual maturation, neurobehavioral changes, and adverse effects on the male reproductive system occurred in the mid- and high-dose groups.
(I)
妊娠した雌は妊娠日 (GD) 6日から17日まで曝露された。高用量群(250 mg/kg体重/日)の雌2匹に、CBD投与に関連した子宮内の同腹の子の全面的な喪失を経験した。高用量群における過剰肝葉のような胎児の構造的変異の発生には対照群と比較して統計学的に有意な差があり、これはヒトで予想されるよりも高い母体血漿中濃度と関連していた。
(II)
雄と雌のラットが交配前2週間からGD 6日まで曝露された。中および高用量群の雄では、全体的な体重増加がより低いことが観察された;雌では体重増加への影響は観察されなかった。中用量群の雄1匹および高用量群の雄1匹は雌を妊娠させることができなかった。剖検では、試験した全用量で雄生殖器重量の減少が認められた。
(III)
妊娠した雌がGD 6日から妊娠および授乳中 (出生後 [PND] 21日まで) 暴露された。ここでは、泌尿生殖器領域の毛の染色が全用量で観察された;中および高用量群では、臍帯付着の発生率の増加、出生児の小型化、妊娠期間の短縮、騒がしい呼吸、発達指標(すなわち、耳介展開、開眼、瞳孔反射)の達成遅延、性成熟の遅延、神経行動学的変化、雄の生殖器系への有害影響がた発生した。
The Epidiolex label states that no adverse effects on fertility in rats occurred after CBD administration prior to and throughout mating and into early gestation. However, it does conclude that administration of CBD to pregnant rats produced evidence of developmental toxicity in the absence of maternal toxicity, at maternal plasma cannabidiol levels greater than that in humans at therapeutic doses. These outcomes include decreased growth; delayed sexual maturation; decreased neurobehavioral activity; and reduction in size and development of the testes in males (FDA, 2018c).
Epidiolexのラベルには、CBD投与後、交配前、交配期間中および妊娠初期まで、ラットの生殖能に対する有害影響は生じなかったと記載されている。しかしながら、妊娠ラットへのCBD投与は、母体毒性がなくても、母体血漿カンナビジオール濃度が治療用量のヒトよりも高い場合に、発生毒性の証拠をもたらしたと結論付けている。これらの成果には、成長の低下;性成熟の遅延;神経行動活動の低下;雄における精巣の大きさ及び発達の減少が含まれる (FDA, 2018c)。
Male reproductive toxicity outcomes are commonly observed following CBD exposure and have been the topic of a recent review article (Carvalho et al., 2020). These effects have been reported across multiple different species, despite inherent interspecies differences in CBD's metabolism and toxicokinetic profile as a whole.
雄の生殖毒性結果はCBD曝露後に一般的に観察され、最近のレビュー論文の話題となっている (Carvalho et al., 2020)。これらの影響は、CBDの代謝および全体としての毒物動態プロファイルに固有の種間差があるにもかかわらず、複数の異なる種にわたって報告されている。
2.1.3. Lagomorphs(ウサギ目)
Nonclinical studies reviewed by FDA for Epidiolex also include an embryofetal development study in New Zealand White rabbits (FDA, 2018b). In this study, mated female rabbits were administered CBD (0, 50, 80, or 125 mg/kg BW/day) by oral gavage throughout organogenesis (GD 7 through 19). Animals were sacrificed on GD 29, at which time pregnancy outcomes were evaluated. Common structural variations indicative of delayed development were more frequently observed in fetuses from the high-dose group (125 mg/kg BW/day). These structural variations appear to be associated with decreased fetal and maternal body weights. The Epidiolex label concludes that developmental toxicity outcomes in rabbits occurred at maternal plasma exposures similar to that in humans at therapeutic doses (FDA, 2018c).
FDAが審査したEpidiolexの非臨床試験には、New Zealand Whiteウサギ(FDA、2018b)を用いた胚胎児発生に関する試験がある。この研究では、交尾した雌ウサギにCBD(0、50、80または125 mg/kg 体重/日)を器官形成期 (GD 7日~19日) を通して強制経口投与した。動物はGD 29日に屠殺され、その時点での妊娠結果を評価された。高用量群(125 mg/kg 体重/日)の胎児では、発達遅延を示す一般的な構造的変異がより頻繁に観察された。これらの構造的変異は胎児および母体の体重減少と関連しているようである。Epidiolexのラベルは、ウサギにおける発生毒性の結果は、治療用量のヒトにおけるものと同様の母体血漿暴露で生じたと結論付けている (FDA, 2018c)。
2.1.4. Phylogenetically distant organisms and in vitro studies(系統学的に遠い生物とin vitro研究)
The trend of CBD-induced male reproductive and developmental toxicity also holds true for organisms phylogenetically distant from humans, such as sea urchins, zebrafish, and chickens, as well as in in vitro studies. In sea urchins (Strongylocentrotus purpuratus), pre-incubation of spermatozoa with THC or CBD inhibited the acrosomal reaction, which is necessary for fertilization (Schuel et al., 1991). Zebrafish exposed to THC or CBD from the blastula stage through the larval stage (96 h post-fertilization) exhibited developmental toxicity, as reported by edemas, curved axis, physical deformities (eye/snout/jaw/trunk/fin), swim bladder distention, and behavioral abnormalities. Importantly, CBD was found to be a more potent developmental toxicant in zebrafish than THC (LC50 for CBD = 0.53 mg/L; LC50 of THC = 3.65 mg/L) (Carty et al., 2019).
CBDによる雄の生殖毒性と発生毒性の傾向は、in vitro試験だけでなく、ウニ、ゼブラフィッシュ、ニワトリなどのヒトから系統的に離れた生物にも当てはまる。ウニ(Strongylocentrotus purpuratus=アメリカムラサキウニ)において、精子をTHCまたはCBDと前培養すると、受精に必要な先体反応が阻害された (Schuel et al., 1991)。胞胚期から幼生期 (受精後96時間) までTHCまたはCBDに曝露されたゼブラフィッシュは、浮腫、湾曲した軸、身体的変形 (眼/鼻/顎/体幹/ヒレ)、浮き袋の膨張、および行動異常によって報告されているように、発生毒性を示した。重要なことに、CBDはゼブラフィッシュにおいてTHC (CBDのLC50=0.53 mg/L;THCのLC50=3.65 mg/L)よりも強力な発生毒性物質であることが分かった(Carty et al., 2019)。
An in ovo study examined the effects of CBD, synthetic cannabinoids (HU 210 and HU 211), and AEA on the viability and development of chick embryos (Gustafsson and Jacobsson, 2019). Fertilized chicken egg yolks were injected on days 1, 4, and 7 with the test compounds or vehicle. After day 9, CBD was demonstrated to be embryotoxic at the highest dose tested (50 μM), reducing the number of viable embryos by 80%. The antioxidant α-tocopherol (a form of vitamin E) protected against CBD's embryotoxic effects, suggesting oxidative stress is involved in mediating this toxicity outcome. This study demonstrated that exposure to CBD during early embryonic development decreases embryo viability, highlighting another potential risk of cannabinoid use during pregnancy.
in ovo研究ではニワトリ胚の生存率と発育に対するCBD、合成カンナビノイド (HU 210とHU 211) とAEAの効果を調べた (Gustafsson and Jacobsson, 2019)。受精卵の卵黄を試験化合物または賦形剤と共に1日目、4日目および7日目に注射した。9日後、CBDは試験した最高用量 (50μM) で胚毒性を示し、生存胚数を80%減少させた。抗酸化物質α‐トコフェロール (ビタミンEの1形態) はCBDの胎児毒性作用から保護し、酸化ストレスがこの毒性結果の仲介に関与することを示唆した。この研究は、初期胚発生中のCBDへの曝露が胚生存率を低下させることを実証し、妊娠中のカンナビノイド使用の別の潜在的リスクを強調した。
In vitro, CBD, and to a lesser extent THC, inhibited testosterone production in rat Leydig cells (Jakubovic et al., 1979; Gorzalka et al., 2010). In human primary and in mouse immortalized Sertoli cells, CBD and its main human metabolites, 7-COOH-CBD and 7-OH-CBD, induced cytotoxicity resultant from the inhibition of the G1/S-phase cell cycle transition, inhibition of DNA synthesis, and downregulation of key cell cycle and functional proteins. Importantly, CBD and its main human metabolites were more cytotoxic to human Sertoli cells than to mouse Sertoli cells (Li et al., 2022). Exogenous CBD exposure in vitro also interfered with cellular processes involved in human placental development and function (Alves et al., 2021), endometrial stromal cell differentiation (Almada et al., 2020), and trophoblast-endometrial crosstalk (Neradugomma et al., 2019); processes necessary for uterine receptivity, implantation, and successful pregnancy. These data demonstrate that the reproductive toxicity of CBD may not be limited to male fertility parameters but could also include effects on female fertility and pregnancy outcomes.
in vitroでは、CBDとそれより程度の低いTHCは、ラットのLeydig細胞におけるテストステロン産生を阻害した(Jakubovic et al., 1979;Gorzalka et al., 2010)。ヒト初代細胞およびマウス不死化のSertoli細胞において、CBDおよびその主要なヒト代謝物である7-COOH-CBDおよび7-OH-CBDは、G1/S期細胞周期移行の阻害、DNA合成の阻害、ならびに鍵となる細胞周期および機能性タンパク質のダウンレギュレーションから生じる細胞毒性を誘発した。重要なことに、CBDとその主要なヒト代謝産物は、マウスSertoli細胞よりもヒトSertoli細胞に対してより細胞毒性であった(Li et al., 2022)。外因性CBDへのin vitro曝露は、ヒト胎盤の発達および機能(Alves et al., 2021)、子宮内膜間質細胞の分化(Almada et al., 2020)、栄養膜-子宮内膜の相互作用(Neradugomma et al., 2019)に関与する細胞プロセスも阻害した;子宮の受容性、着床、妊娠の成功に必要なプロセス。これらのデータは、CBDの生殖毒性が男性の受胎能パラメータに限定されず、女性の受胎能および妊娠結果への影響も含む可能性があることを示している。
In total, there are clear reproductive toxicity effects following CBD exposure in adult males and gestational CBD exposure in male offspring, specifically on spermatogenesis, which can result in abnormal sperm morphology and the subsequent prevention of oocyte fertilization. In concordance with CBD's male reproductive toxicity across phylogenetically distant species, developmental toxicity effects were frequently observed across animal models (FDA, 2018b).
全体として、成人男性におけるCBD暴露、および男性の子孫における妊娠中のCBD暴露には、明確な生殖毒性、特に精子形成に対する影響があり、精子の形態異常およびその後の卵母細胞受精の阻害を引き起こす可能性がある。系統発生的に遠い種を越えたCBDの雄生殖毒性と一致して、発生毒性影響は動物モデルを越えて頻繁に観察された(FDA, 2018b)。
2.2. Hepatotoxicity(肝毒性)
Hepatotoxic effects of orally administered CBD, such as increased liver weights and elevated liver enzymes, have been reported in both animal and human studies. In clinical trials for the CBD-based drug Epidiolex, elevated liver enzymes were observed in 5–20% of epileptic patients treated with CBD, and a few patients were withdrawn for having elevated liver aminotransferase enzymes that exceeded 3 times the upper limit of normal (Devinsky et al., 2017, 2018a; Thiele et al., 2018). The majority of epileptic patients who presented with elevated liver enzymes were also concomitantly prescribed other anti-epileptic medications, like valproate, which itself carries a risk for elevating liver aminotransferases. However, in a more recent publication, elevated liver enzymes were also noted in healthy adults given CBD, who were not on other medications at the time. These findings suggest that CBD consumption could also cause drug-induced liver injury in non-epileptic individuals (Watkins et al., 2021).
肝重量の増加および肝酵素の上昇など、経口投与されたCBDの肝毒性作用が、動物およびヒトの両方の研究で報告されている。CBDベースの薬剤であるEpidiolexの臨床試験では、CBDを投与されたてんかん患者の5%~20%に肝酵素の上昇が認められ、肝アミノトランスフェラーゼ値が正常値の上限の3倍を超えたために数名の患者が脱落した(Devinsky et al., 2017, 2018a;Thiele et al., 2018)。肝酵素上昇を呈したてんかん患者の大半は、バルプロ酸などの他の抗てんかん薬も同時に処方されていた、バルプロ酸自体に肝アミノトランスフェラーゼ上昇のリスクがある。しかしながら、より最近の発表では、CBDを投与され、その時点で他の薬剤を投与されていなかった健常成人においても肝酵素の上昇が認められた。これらの知見は、CBD消費が非てんかん患者においても薬物誘発性肝障害を引き起こす可能性があることを示唆している(Watkins et al., 2021)。
In mice, a two-part acute (24 h) and subacute (10-day) exposure study was performed using CBD-rich cannabis extract (CRCE; ∼58% CBD) administered by gavage (Ewing et al., 2019a). In the acute study, the authors chose a single dose of 246, 738 or 2460 mg CRCE/kg BW/day. Results reported a dose-dependent, statistically significant increase in both liver enzymes aspartate aminotransferase (AST) and alanine aminotransferase (ALT), as well as a marked elevation of total bilirubin at the highest dose; indicating that CBD can cause a dose-dependent increase in hepatotoxicity. In the 10-day study, doses of 61.5, 184.5, and 615 mg/kg BW/day for ten non-consecutive days were used. The lowest dose of 61.5 mg/kg BW was determined equivalent to a human CBD dose of 5 mg/kg BW, the recommended daily starting dosage of Epidiolex, based on the CBD content of the extract and the use of allometric scaling between mouse and human. Histopathological evaluation revealed pan-hepatic cytoplasmic swelling at the highest dose (615 mg/kg BW), with foci of cytoplasmic swelling in the mid-dose (184.5 mg/kg BW) group. At the lowest dose (61.5 mg/kg BW), an increase in the relative liver and kidney weights, and increases in ALT, AST, and serum bilirubin were reported. Additionally, in both parts of the study, gene expression analysis revealed a dose-dependent increase in several CYP and UGT isoforms. This study suggests that oral exposure to CBD through CRCE, at a therapeutically relevant dose, can result in overt signs of hepatotoxicity.
マウスでは、強制経口によって投与されるCBDに富む大麻抽出物(CRCE;~58% CBD)を用いて、急性 (24時間) および亜急性 (10日間) の2部構成の曝露試験が実施された(Ewing et al., 2019a)。急性試験では、著者らはCRCE 246、738 mg/kg 体重/日または2460 mg/kg体重/日の単回投与を選択した。その結果は、肝臓酵素のアスパラギン酸アミノトランスフェラーゼ (AST) およびアラニンアミノトランスフェラーゼ (ALT) が用量依存的に統計学的に有意に増加し、最高用量で総ビリルビンが著しく上昇したことを報告した;CBDは用量依存的に肝毒性を増加させる可能性があることを示している。10日間の試験では、61.5、184.5および615 mg/kg 体重/日を非連続10日間投与した。抽出物のCBD含量とマウスとヒトの間の相対成長スケーリングの使用に基づいて、61.5 mg/kg 体重の最低用量はEpidiolexの推奨1日開始用量である5 mg/kg 体重のヒトCBD用量に相当すると決定された。組織病理学的評価は、最高用量 (615 mg/kg 体重) で汎肝細胞質腫脹を明らかにし、中用量 (184.5 mg/kg 体重) 群で細胞質腫脹巣を明らかにした。最低用量 (61.5 mg/kg体重) では、肝臓と腎臓の相対重量の増加、ALT、AST、血清ビリルビンの増加が報告された。さらに、研究の両部分において、遺伝子発現解析は、いくつかのCYPおよびUGTアイソフォームの用量依存的増加を明らかにした。この研究は、治療的に適切な用量でCRCEを介したCBDへの経口曝露が肝毒性の明白な徴候をもたらす可能性があることを示唆する。
The same researchers conducted another study in mice to investigate liver effects following exposure to a combination of CRCE and a common over the counter anti-inflammatory medication, acetaminophen (APAP) (Ewing et al., 2019b). APAP is known to cause liver injury through glutathione depletion and oxidative stress. In this study, female mice were orally administered CRCE at doses of 116 or 290 mg/kg BW/day for three consecutive days, followed by an intraperitoneal challenge with APAP. The lower dose (116 mg/kg BW + APAP) produced liver injury (i.e., sinusoidal obstruction) and mortality. These effects were not observed in the control APAP group. Paradoxically, in the high-dose group (290 mg/kg BW + APAP), no mortality was observed, and liver injury was noted in only 25% of mice. The lower incidence of hepatotoxicity in the 290 mg/kg BW group was associated with a rapid re-synthesis of glutathione such that the total glutathione level was similar to that in controls. These findings highlight the potential for CBD-drug interactions as revealed by the paradoxical effect of the combination of CBD and APAP. This is consistent with other evidence suggesting that CBD can interact with drugs and lead to serious adverse health effects, including liver injury (Devinsky et al., 2018b).
同じ研究者らは、マウスを用いた別の試験を実施し、CRCEと一般的な市販抗炎症薬であるアセトアミノフェン (APAP)の併用曝露後の肝臓への影響を調べた (Ewing et al., 2019b)。APAPはグルタチオン枯渇と酸化ストレスを介して肝損傷を引き起こすことが知られている。この研究では、雌マウスにCRCEを116または290 mg/kg 体重/日の用量で3日連続経口投与し、続いてAPAPを腹腔内投与した。低用量 (116 mg/kg BW+APAP) は肝臓損傷(すなわち肝中心静脈閉塞)と死亡を生じた。これらの効果は対照APAP群では観察されなかった。逆説的であるが、高用量群 (290 mg/kg BW+APAP) では、死亡は観察されず、肝損傷はマウスのわずか25%に認められた。290 mg/kg 体重群における肝毒性の低い発生率は、総グルタチオンレベルが対照群と類似するようなグルタチオンの迅速な再合成と関連していた。これらの知見は、CBDとAPAPの併用の逆説的効果によって明らかにされるCBD‐薬物相互作用の可能性を強調する。これは、CBDが薬物と相互作用し、肝障害を含む重篤な有害な健康影響を引き起こす可能性があることを示唆する他の証拠と一致している (Devinsky et al., 2018b)。
For the CBD-based drug Epidiolex, a 26-week oral toxicity study in rats and a 39-week oral toxicity study in dogs were conducted (FDA, 2018b). In rats, centrilobular hypertrophy was observed in the mid-dose (50 mg/kg BW) and high-dose (150 mg/kg BW) groups in both sexes, and was associated with increased ALT, ALP, and relative liver weights. In dogs, decreases in absolute body weight were observed at all doses (low-10 mg/kg BW, mid-50 mg/kg BW, and high-dose 100 mg/kg BW) in both sexes. Hepatocellular hypertrophy was also detected at all doses in both sexes, coupled with a slight increase in ALT and an 8-fold increase in ALP. The observed liver hypertrophy was associated with increased liver weights and macroscopic enlargement. Additionally, a decreased heart rate was observed in high-dose males only.
CBD製剤であるEpidiolexについて、ラットで26週間の経口投与毒性試験及びイヌで39週間の経口投与毒性試験が実施された(FDA, 2018b)。ラットでは、小葉中心性の肥大が雌雄の中用量 (50 mg/kg体重) および高用量 (150 mg/kg体重) 群で観察され、ALT、ALPおよび相対肝重量の増加と関連していた。イヌでは、雌雄ともに全用量(低用量10 mg/kg 体重、中間用量50 mg/kg 体重、高用量100 mg/kg 体重)で絶対体重の減少が認められた。肝細胞肥大も雌雄の全用量で認められ、ALTのわずかな増加およびALPの8倍の増加も認められた。観察された肝肥大は、肝重量の増加および肉眼的肥大と関連していた。さらに、心拍数の減少が高用量の雄のみに認められた。
These studies clearly establish that orally consumed CBD has the potential to elicit hepatotoxic effects in rodents, dogs, and humans, as evidenced by increases in various biomarkers of hepatotoxicity, like liver enzymes and bilirubin, and histopathological changes.
これらの研究は、肝酵素およびビリルビンのような肝毒性の種々のバイオマーカーの増加および組織病理学的変化によって証明されるように、経口摂取されたCBDがげっ歯類、イヌおよびヒトにおいて肝毒性効果を誘発する可能性があることを明確に立証する。
2.3. Immunotoxicity(免疫毒性)
CBD has been shown to suppress the immune function of splenocytes (T and B lymphocytes) directly exposed in vitro or isolated from CBD-exposed mice. Direct exposure caused these cells to become apoptotic in a concentration dependent manner after 12 h of exposure (Wu et al., 2008).
CBDは、in vitroで直接曝露された、またはCBD曝露マウスから単離された脾臓細胞 (TおよびBリンパ球) の免疫機能を抑制することが示されている。直接曝露は、12時間の曝露後にこれらの細胞を濃度依存的にアポトーシスを起こした (Wu et al., 2008)。
Similarly, CBD induced apoptosis in normal murine thymocytes (T lymphocytes) and in EL-4 thymoma cells (Lee et al., 2008). Here, the induction of apoptosis occurred in a time- and concentration-dependent manner in both cell types, with a 50% effective concentration (EC50) of about 7 μM in normal murine thymocytes. This work demonstrates that normal thymocytes are more sensitive to CBD-induced damage than thymoma cells in vitro. Further investigation revealed that the cellular damage was caused by reactive oxygen species and oxidative stress induced by CBD treatment. In an attempt to reverse the CBD-induced oxidative stress, Lee et al. co-treated thymocytes with CBD and N-acetyl-L-cysteine, a glutathione precursor, which was able to attenuate this effect. These results are consistent with the similar findings in splenic lymphocytes (Wu et al., 2008) and leukemia cells (McKallip et al., 2006).
同様に、CBDは正常マウス胸腺細胞 (Tリンパ球) およびEL‐4胸腺腫細胞においてアポトーシスを誘導した (Lee et al., 2008)。アポトーシスの誘発は両細胞型で時間及び濃度依存的に起こり、正常マウス胸腺細胞における50%有効濃度 (EC50) は約7μMであった。この研究は、正常胸腺細胞がin vitroで胸腺腫細胞よりもCBD誘発性の損傷に対して感受性が高いことを示す。さらなる研究は、細胞損傷がCBD処理により誘発される活性酸素種および酸化ストレスにより引き起こされることを明らかにした。CBD誘発性の酸化ストレスを逆転させる試みにおいて、Leeらは胸腺細胞をCBDおよびグルタチオン前駆体であるN-アセチル-L-システインと共処理し、この作用を減弱させることができた。これらの結果は、脾臓リンパ球(Wu et al., 2008)および白血病細胞(McKallip et al., 2006)における同様の所見と一致した。
Several in vitro studies and one in vivo study suggest that CBD can induce damage to both normal and cancer-derived lymphatic cells. These findings suggest oxidative stress caused by a reduction in the level of intracellular glutathione and apoptosis caused by the activation of multiple caspases may be involved in the immunotoxicity potential of CBD.
いくつかのin vitro研究と1件のin vivo研究は、CBDが正常および癌由来リンパ細胞の両方に損傷を誘発することを示唆している。これらの知見は、細胞内グルタチオンレベルの低下に起因する酸化ストレスおよび複数のカスパーゼの活性化に起因するアポトーシスが、CBDの免疫毒性能力に関与する可能性を示唆する。
In support of the potential for CBD to suppress immune cell function, clinical studies on the CBD-based drug Epidiolex show a potential dose-dependent increase in infections, particularly pneumonia (FDA, 2018d).
CBDが免疫細胞機能を抑制する可能性を裏付けるものとして、CBDベースの薬剤であるEpidiolexに関する臨床試験では、感染症、特に肺炎が用量依存的に増加する可能性が示されている (FDA, 2018d)。
2.4. Mutagenicity and genotoxicity(変異原性と遺伝毒性)
The genotoxicity potential of CBD has been reported in several published studies with conflicting conclusions as reviewed and discussed below.
CBDの遺伝毒性の可能性は、いくつかの公表された研究で報告されており、以下に概説し考察するように、結論は矛盾している。
Genotoxicity assays conducted on the CBD-based drug Epidiolex, inclusive of Ames assay, in vivo micronucleus assay in rat, and in vivo alkaline COMET assay, reported that CBD is non-mutagenic and non-genotoxic under the conditions of the assays (FDA, 2018b). Additionally, in a double-blind placebo-controlled study in humans, there were no statistically significant changes in chromosome damage after administering 1200 mg CBD/day to study volunteers for 20 consecutive days (Matsuyama and Fu, 1981).
CBDベースの薬剤Epidiolexについて実施された遺伝毒性試験は、Ames試験、ラットにおけるin vivo小核試験、およびin vivoアルカリ性COMET試験を含み、CBDは試験条件下で非変異原性および非遺伝毒性であることが報告された (FDA, 2018b)。さらに、ヒトにおける二重盲検プラセボ対照試験では、被験者にCBD 1200 mg/日を20日間連続投与しても、染色体損傷に統計的に有意な変化はみられなかった (Matsuyama and Fu, 1981)。
However, in mice, intraperitoneal administration of 10 mg/kg BW/day of CBD for 5 consecutive days produced statistically significant increases in the percentage of micronuclei in bone marrow polychromatic erythrocytes compared to controls, with a noted increase in the incidence of chromosomal abnormalities (Zimmerman and Raj, 1980). Additionally, exposure of human-derived HepG2 and TR146 cells to highly purified CBD resulted in single and double-stranded DNA breaks (Russo et al., 2019).
しかしながら、マウスに10 mg/kg 体重/日のCBDを連続5日間腹腔内投与したところ、対照群と比較して骨髄多染性赤血球中の小核の割合が統計的に有意に増加し、染色体異常の発生率が顕著に増加した (Zimmerman and Raj, 1980)。さらに、ヒト由来HepG2細胞およびTR146細胞を高度に精製したCBDに曝露すると、一本鎖および二本鎖DNA切断が生じた (Russo et al., 2019)。
Conflicting reports on CBD's potential genotoxicity and clastogenicity have been previously reviewed (Zimmerman and Zimmerman, 1990). The reviewers opined that the conflicting reports on the cytogenetic effects can be partially explained by the different experimental protocols, cell types, and/or the animal species used by the investigators.
CBDの潜在的遺伝毒性および染色体異常誘発性に関する相反する報告が以前にレビューされている(Zimmerman and Zimmerman, 1990)。審査官は、細胞遺伝学的影響に関する矛盾した報告は、研究者らが用いた実験プロトコル、細胞型および/または動物種の違いによって部分的に説明できると考えた。
2.5. Inhibition of Drug/Xenobiotic Metabolizing Enzymes and Transport Proteins, and CBD-Drug Interaction Potential(薬物/異物代謝酵素および輸送タンパク質の阻害、および CBD と薬物の相互作用の可能性)
In animal models, CBD has been shown to inhibit hepatic microsomal drug metabolism through inhibition of specific CYP enzymes (Paton and Pertwee, 1972), based on the observation in mice that pentobarbitone-induced sleep was significantly prolonged by CBD treatment in a dose-dependent manner. This suggests that CBD-induced inhibition of microsomal enzymes prevented pentobarbitone metabolism. An additional study in mice reported that CBD treatment resulted in a decrease in testosterone 6β-hydroxylation and erythromycin N-demethylation; both reactions are markers of CYP3A activity, indicating that CBD could inhibit CYP3A (Yamamoto et al., 1995). As with mice, in male rats, CBD inhibited CYP2C11 function, resulting in decreased 2α- and 16α-hydroxylation of testosterone (Narimatsu et al., 1988). Interestingly, work by other authors in rats demonstrated that CBD may disrupt hormone homeostasis and steroidogenesis through selective inhibition of male specific CYPs, like CYP2C11, in the liver (Watanabe et al., 2005).
動物モデルにおいて、CBDは、ペントバルビトン誘導睡眠がCBD処理により用量依存的に有意に延長されたというマウスの観察に基づき、特異的CYP酵素の阻害を介して肝臓ミクロソーム薬物代謝を阻害することが示されている (Paton and Pertwee, 1972)。これは、ミクロソーム酵素のCBD誘導阻害がペントバルビトン代謝を阻害することを示唆する。マウスを用いた追加試験では、CBD投与によりテストステロン6β-水酸化およびエリスロマイシンN-脱メチル化が減少したことが報告されている;いずれの反応もCYP3A活性のマーカーであり、CBDがCYP3Aを阻害する可能性が示された (Yamamoto et al., 1995)。マウスと同様に、雄ラットにおいて、CBDはCYP2C11機能を阻害し、テストステロンの2α-および16α-水酸化を低下させた(Narimatsu et al., 1988)。興味深いことに、他の著者らによるラットを用いた研究では、CBDが肝臓におけるCYP2C11のような男性の特異的CYPの選択的阻害を介してホルモンの恒常性とステロイド産生を阻害する可能性があることが実証された(Watanabe et al., 2005)。
An in vitro study using human liver microsomes showed that CBD is a potent inhibitor of specific human CYPs: CYP1A1, CYP2B6, CYP2C9, CYP2C19, CYP2D6, CYP3A4 and CYP3A5 (Jiang et al., 2011). Importantly, a study associated with the CBD-based drug Epidiolex reported that CBD can inhibit (IC50 < 10 mM) the function of multiple CYP isoforms in vitro, including CYP1A2, CYP2B6, CYP2C8, CYP2C19, and CYP3A4 (FDA, 2018a). These data suggest CBD may compete with pharmaceuticals and xenobiotics that use a similar metabolism pathway; in turn, raising the possibility for drug-drug interactions.
ヒト肝臓ミクロソームを用いたin vitro試験では、CBDは特定のヒトCYPの強力な阻害剤であることが示された:CYP1A1、CYP2B6、CYP2C9、CYP2C19、CYP2D6、CYP3A4およびCYP3A5 (Jiang et al., 2011)。重要なことに、CBDベースの薬剤Epidiolexに関連する研究は、CBDがin vitroでCYP1A2、CYP2B6、CYP2C8、CYP2C19、CYP3A4 を含む複数のCYPアイソフォームの機能を阻害できる(IC50<10 mM)ことを報告した (FDA, 2018a)。これらのデータは、CBDが類似の代謝経路を用いる医薬品および生体異物と競合する可能性を示唆している;その結果、薬物間相互作用の可能性が高まります。
In addition to inhibiting CYP enzymes, CBD can also inhibit crucial efflux transporters like P-gp and BCRP. In vitro studies have shown that CBD (3–100 μM) can inhibit P-gp mediated efflux, leading to an increased intracellular accumulation of undesirable P-gp substrates, like phenobarbital and digoxin (Iffland and Grotenhermen, 2017). Additionally, in an in vitro model of the placental barrier using choriocarcinoma BeWo and Jar cells, both of which express the efflux transporter BCRP, exposure to 10 and 25 μM CBD resulted in a dose-dependent accumulation of mitoxantrone, a BCRP substrate, in both cell types (Feinshtein et al., 2013). This demonstrated that CBD inhibits BCRP-mediated efflux of mitoxantrone. CBD's inhibitory effects on efflux transporters like P-gp and BCRP can cause aberrant accumulation of various compounds, such as xenobiotics and therapeutic drugs, that would otherwise be transported out of the cell. Greater penetration and accumulation of these chemicals in the brain and other tissues raises safety concerns for the general population. It is also important to note that P-gp and BCRP play critical roles in placenta-mediated efflux of pharmaceuticals and xenobiotics. Dysregulation of this fetal protective mechanism by CBD raises safety concerns regarding gestational exposure to CBD in the presence of other pharmacologically active agents or environmental toxicants.
CYP酵素の阻害に加えて、CBDはP‐gpおよびBCRPのような重要な排出輸送体も阻害することができる。In vitro研究では、CBD (3–100 μM)が100 P-gpを介した排出を阻害し、フェノバルビタールやジゴキシンのような望ましくないP-gp基質の細胞内蓄積を増加させることが示された (Iffland and Grotenhermen, 2017)。さらに、排出輸送体BCRPを発現する絨毛がんBeWo細胞およびJar細胞を用いた胎盤関門のin vitroモデルにおいて、10および25μMのCBDに曝露すると、両細胞型においてBCRP基質であるミトキサントロンが用量依存的に蓄積した(Feinshtein et al., 2013)。これは、CBDがBCRPが仲介するミトキサントロンの排出を阻害することを示した。P‐gpやBCRPのような排出輸送体に対するCBDの阻害作用は、そうでなければ細胞外に輸送されるであろう生体異物や治療薬のような種々の化合物の異常な蓄積を引き起こす。脳および他の組織におけるこれらの化学物質のより大きな浸透および蓄積は、一般集団に対する安全性の懸念を引き起こす。P-gpとBCRPが胎盤を介した医薬品と生体異物の排出に重要な役割を果たしていることに注目することも重要である。CBDによるこの胎児保護機構の調節不全は、他の薬理学的活性物質または環境毒性物質の存在下でのCBDへの妊娠曝露に関する安全性の懸念を提起する。
3. Conclusions
The studies and data reviewed herein show potential hazards associated with oral exposure to CBD for the general population. Observed effects include organ weight alterations; developmental and reproductive toxicities in both males and females, including effects on neuronal development and embryo-fetal mortality; hepatotoxicity; immune suppression, including lymphocytotoxicity; mutagenicity and genotoxicity; and effects on liver metabolizing enzymes and drug transport proteins.
ここでレビューした研究とデータは、一般集団に対するCBDの経口暴露に関連する潜在的な危険性を示している。観察された影響には、臓器重量の変化;神経発生及び胚と胎児死亡率への影響を含む、男性と女性両方における発生および生殖毒性;肝毒性;リンパ球毒性を含む免疫抑制;変異原性と遺伝毒性;肝代謝酵素及び薬物輸送蛋白への影響を含む。
CBD can cause adverse effects on the male reproductive system from exposure during gestation or adulthood. These effects have been attributed to dysregulated endocannabinoid-modulated steroidogenesis and/or dysregulated hormonal feedback mechanisms, primarily involving testosterone. Available data indicate additional concerns for developmental effects, and suggest the reproductive toxicity of CBD includes female- and pregnancy-specific outcomes. Toxicities observed from gestational exposure to CBD in both sexes, such as delayed sexual maturity, increased pre-implantation loss, and undesirable alterations to the brain epigenome are of particular concern, as these effects could be transgenerational.
CBDは、妊娠中または成人期の曝露により、男性の生殖系に有害影響を及ぼす可能性がある。これらの作用は、主にテストステロンが関与する、内因性カンナビノイド調節ステロイド産生および/またはホルモンフィードバック機構の調節不全に起因している。入手可能なデータは、発生への影響に関するさらなる懸念を示しており、CBDの生殖毒性には女性および妊娠に特異的な結果が含まれることを示唆している。性成熟の遅延、着床前損失の増加および脳エピゲノムへの望ましくない変化のような、両性におけるCBDへの妊娠曝露から観察された毒性は、これらの影響が世代を超えている可能性があるため、特に懸念される。
CBD can also cause adverse effects on the liver. These findings highlight the potential for CBD-drug interactions as revealed by the effect of CBD on multiple drug metabolizing enzymes, and the paradoxical effect of the combination of CBD and APAP. While the impact of CBD on drug metabolizing enzymes is well established, further studies would be needed to investigate the mechanism of CBD's paradoxical interaction with APAP and similar pharmaceuticals.
CBDは肝臓に有害作用を引き起こすこともある。これらの知見は、複数の薬物代謝酵素に対するCBDの効果によって明らかにされるCBD‐薬物相互作用の可能性と、CBDとAPAPの併用の逆説的効果を強調する。薬物代謝酵素に対するCBDの影響は十分に確立されているが、APAPおよび類似の医薬品とのCBDの逆説的相互作用のメカニズムを調査するためにはさらなる研究が必要であろう。
The diverse and disparate effects observed following CBD exposure suggest multiple potential mechanisms of toxicity. Analysis of identified CBD cellular targets and their native functions suggests the following possible mechanisms of CBD-mediated toxicity: (I) inhibition of, or competition for, several metabolic pathway enzymes, including both phase I and II drug metabolizing enzymes, (II) receptor binding activity, (III) disruption of testosterone steroidogenesis, (IV) inhibition of the reuptake and breakdown of endocannabinoids, and (V) oxidative stress via depletion of cellular glutathione in the liver or inhibition of testicular enzymatic activity. CBD may additionally act though secondary mechanisms to impact reproduction and development. For instance, CBD was shown in vitro to inhibit TRPV1, dysregulation of which has been observed in placentas from preeclamptic pregnancies (Martinez et al., 2016).
CBD暴露後に観察された多様で異なる影響は、複数の潜在的な毒性メカニズムを示唆している。同定されたCBD細胞標的およびそれらの本来の機能の分析は、以下のCBD媒介毒性の可能性のあるメカニズムを示唆している:(I) 第I相および第II相薬物代謝酵素の両方を含むいくつかの代謝経路酵素の阻害または競合、(II) 受容体結合活性、(III) テストステロンステロイド合成の破壊、(IV) 内因性カンナビノイドの再取り込みおよび分解の阻害、および (V) 肝臓における細胞グルタチオンの枯渇または精巣酵素活性の阻害を介した酸化ストレス。CBDはさらに、生殖と発生に影響を与える二次的メカニズムを介して作用する可能性がある。例えば、CBDはin vitroでTRPV1を阻害することが示されており、その調節不全は子癇前症の妊娠の胎盤で観察されている(Martinez et al., 2016)。
Although CBD's mechanisms of action remain unclear and are likely multifarious, many proposed mechanisms relate to the endocannabinoid system. Physiological processes controlled by the endocannabinoid system are areas of potential concern for CBD toxicity. It bears noting that the endocannabinoid system is still poorly understood, and future elucidation of its intricacies may provide new insight into safety concerns for perturbation of this biological system and the mechanisms of CBD's effects. Demonstrated differences between THC's and CBD's biological effects and toxicities highlights the complexity of this system. While this review focuses on relatively pure CBD, many other phytocannabinoids with structural similarity to CBD exist for which there is little or no toxicological data to evaluate their safety.
CBDの作用機序は不明のままであり、おそらく多種多様であるが、提案されている機序の多くは内因性カンナビノイド系に関連している。内因性カンナビノイドシステムによって制御される生理学的プロセスは、CBD毒性の潜在的な懸念事項である。内因性カンナビノイドシステムはまだ十分に理解されておらず、その複雑さの将来の解明は、この生物学的システムの混乱に対する安全性の懸念とCBDの効果のメカニズムに新しい洞察を提供する可能性があることを留意する。THCとCBDの生物学的効果と毒性の間の実証された違いは、このシステムの複雑さを強調する。本レビューは比較的純粋なCBDに焦点を当てているが、CBDと構造的に類似した多くの他の植物性カンナビノイドが存在し、それらの安全性を評価するための毒性学的データはほとんどまたは全くない。
Potential adverse effects from CBD use may not be immediately evident to users of CBD-containing consumer products. For example, early signs of liver toxicity would go undetected without monitoring for such effects. Additionally, effects observed on the male reproductive system in animal models involve damage to testicular structure and function, including effects on the development and abundance of spermatozoa, in the absence of any outwardly visible damage. If these effects are relevant to humans, they imply that chronic consumption of CBD could interfere with male reproductive function in a way that may only manifest as a reduction, or non-recurrent failure, in reproductive success (i.e., subfertility). Thus, it would be difficult to identify such outcomes through typical post-market monitoring and adverse event reporting systems.
CBD使用による潜在的な有害影響は、CBDを含む消費者向け製品の使用者にはすぐには明らかにならないかもしれない。例えば、肝臓毒性の初期徴候は、そのような影響のモニタリングなしには検出されないであろう。さらに、動物モデルで観察された雄の生殖系への影響には、外観上の損傷がなくても、精子の発生と量への影響を含む精巣の構造と機能への損傷が含まれる。これらの影響がヒトに関連しているとすれば、CBDの慢性的な摂取は、生殖成功率の低下または非再発性の失敗(すなわち、低受胎)としてのみ現れる方法で、男性の生殖機能に干渉する可能性があることを示唆している。したがって、一般的な市販後モニタリングや有害事象報告システムを通じてそのような結果を特定することは困難であろう。
The available data clearly establish CBD's potential for adverse health effects when consumed without medical supervision by the general population. Some risks, such as the potential for liver injury, will likely be further characterized with ongoing clinical observations. Other observed effects from the toxicology data, such as male and potential female reproductive effects, have not been documented in humans but raise significant concerns for the use of CBD (in oral consumer products) by the broad population. Importantly, the degree of reproductive effects and the wide range of species impacted further contributes to the concerns around CBD consumption by the general population.
入手可能なデータは、一般集団が医学的な監督なしに消費した場合のCBDの健康への悪影響の可能性を明確に立証している。肝臓障害の可能性のようないくつかのリスクは、進行中の臨床観察によってさらに特徴づけられるであろう。毒性データから観察されたその他の影響、例えば男性および潜在的な女性の生殖への影響はヒトでは報告されていないが、CBD (経口消費者製品) の広範な集団による使用には重大な懸念が生じる。重要なことに、生殖への影響の程度と影響を受ける広範な種は、一般集団によるCBD消費に関する懸念にさらに寄与している。
Adverse health effects have been observed in humans and animals at levels of intake that could reasonably occur from the use of CBD-containing consumer products (Dubrow et al., 2021). CBD's lengthy t1/2 following chronic oral administration makes long-term consumption of CBD products by the broad population concerning. Available data from multiple oral toxicity studies raise serious safety questions about the potential for reproductive and developmental toxicity effects, which could be irreversible, and support particular concerns about the use of CBD during pregnancy or in combination with other drugs.
健康への悪影響は、CBDを含む消費者製品の使用から合理的に発生する可能性のある摂取量で、ヒトおよび動物で観察されている (Dubrow et al., 2021)。慢性経口投与後のCBDの長いt1/2は、広範な集団によるCBD製品の長期消費を懸念させる。複数の経口毒性試験から得られた利用可能なデータは、不可逆的な可能性のある生殖および発生毒性影響の可能性について重大な安全性の疑問を提起し、妊娠中または他の薬剤との併用におけるCBDの使用に関する特別な懸念を支持する。
Funding
This work received no grants from any funding agency in the public, commercial, private, or not-for-profit sectors.
CRediT authorship contribution statement
Jeremy Gingrich: Investigation, Writing – original draft, Writing – review & editing. Supratim Choudhuri: Conceptualization, Investigation, Writing – original draft. Patrick Cournoyer: Conceptualization, Writing – review & editing, Project administration. Jason Downey: Writing – review & editing. Kristi Muldoon Jacobs: Writing – review & editing.
Declaration of competing interest
The authors declare that they have no known competing financial interests or personal relationships that could have appeared to influence the work reported in this paper.
Data availability
No data was used for the research described in the article.
References
Aizpurua-Olaizola et al., 2016
O. Aizpurua-Olaizola, et al.
Evolution of the cannabinoid and terpene content during the growth of cannabis sativa plants from different chemotypes
J. Nat. Prod., 79 (2) (2016), pp. 324-331
Crossref View in Scopus Google Scholar
Almada et al., 2020
M. Almada, et al.
Cannabidiol (CBD) but not tetrahydrocannabinol (THC) dysregulate in vitro decidualization of human endometrial stromal cells by disruption of estrogen signaling
Reprod. Toxicol., 93 (2020), pp. 75-82
View PDF View article View in Scopus Google Scholar
Alves et al., 2021
P. Alves, et al.
Cannabidiol disrupts apoptosis, autophagy and invasion processes of placental trophoblasts
Arch. Toxicol., 95 (10) (2021), pp. 3393-3406
View at publisher Crossref View in Scopus Google Scholar
Barrie and Manolios, 2017
N. Barrie, N. Manolios
The endocannabinoid system in pain and inflammation: its relevance to rheumatic disease
Eur. J. Rheumatol., 4 (3) (2017), pp. 210-218
View at publisher Crossref Google Scholar
Bisogno et al., 2001
T. Bisogno, et al.
Molecular targets for cannabidiol and its synthetic analogues: effect on vanilloid VR1 receptors and on the cellular uptake and enzymatic hydrolysis of anandamide
Br. J. Pharmacol., 134 (4) (2001), pp. 845-852
Crossref View in Scopus Google Scholar
Bloch et al., 1978
E. Bloch, et al.
Effects of cannabinoids on reproduction and development
Vitam. Horm., 36 (1978), pp. 203-258
Google Scholar
Carty et al., 2019
D.R. Carty, et al.
Multigenerational consequences of early-life cannabinoid exposure in zebrafish
Toxicol. Appl. Pharmacol., 364 (2019), pp. 133-143
View PDF View article View in Scopus Google Scholar
Carvalho et al., 2018a
R.K. Carvalho, et al.
Chronic cannabidiol exposure promotes functional impairment in sexual behavior and fertility of male mice
Reprod. Toxicol., 81 (2018), pp. 34-40
View PDF View article View in Scopus Google Scholar
Carvalho et al., 2018b
R.K. Carvalho, et al.
Chronic exposure to cannabidiol induces reproductive toxicity in male Swiss mice
J. Appl. Toxicol., 38 (9) (2018), pp. 1215-1223
Crossref View in Scopus Google Scholar
Carvalho et al., 2020
R.K. Carvalho, M.L. Andersen, R. Mazaro-Costa
The effects of cannabidiol on male reproductive system: a literature review
J. Appl. Toxicol., 40 (1) (2020), pp. 132-150
Crossref View in Scopus Google Scholar
Carvalho et al., 2022
R.K. Carvalho, et al.
Decreasing sperm quality in mice subjected to chronic cannabidiol exposure: new insights of cannabidiol-mediated male reproductive toxicity
Chem. Biol. Interact., 351 (2022), Article 109743
View PDF View article View in Scopus Google Scholar
Cherniakov et al., 2017
I. Cherniakov, et al.
Piperine-pro-nanolipospheres as a novel oral delivery system of cannabinoids: pharmacokinetic evaluation in healthy volunteers in comparison to buccal spray administration
J. Contr. Release, 266 (2017), pp. 1-7
View PDF View article View in Scopus Google Scholar
Congress, 2017-2018
U. Congress
H.R.2 - 115th congress
Agriculture Improvement Act of 2018. 2018 25 January 2022]; Available from:
https://www.congress.gov/bill/115th-congress/house-bill/2 (2017-2018)
Google Scholar
Cunha et al., 1980
J.M. Cunha, et al.
Chronic administration of cannabidiol to healthy volunteers and epileptic patients
Pharmacology, 21 (3) (1980), pp. 175-185
Crossref View in Scopus Google Scholar
Dalterio, 1980
S.L. Dalterio
Perinatal or adult exposure to cannabinoids alters male reproductive functions in mice
Pharmacol. Biochem. Behav., 12 (1) (1980), pp. 143-153
View PDF View article View in Scopus Google Scholar
Dalterio and deRooij, 1986
S.L. Dalterio, D.G. deRooij
Maternal cannabinoid exposure. Effects on spermatogenesis in male offspring
Int. J. Androl., 9 (4) (1986), pp. 250-258
Crossref View in Scopus Google Scholar
Dalterio et al., 1982
S. Dalterio, et al.
Cannabinoids in male mice: effects on fertility and spermatogenesis
Science, 216 (4543) (1982), pp. 315-316
Crossref View in Scopus Google Scholar
Deutsch, 2016
D.G. Deutsch
A personal retrospective: elevating anandamide (AEA) by targeting fatty acid amide hydrolase (FAAH) and the fatty acid binding proteins (FABPs)
Front. Pharmacol., 7 (2016), p. 370
Crossref View in Scopus Google Scholar
Devinsky et al., 2017
O. Devinsky, et al.
Trial of cannabidiol for drug-resistant seizures in the Dravet syndrome
N. Engl. J. Med., 376 (21) (2017), pp. 2011-2020
Crossref View in Scopus Google Scholar
Devinsky et al., 2018a
O. Devinsky, et al.
Effect of cannabidiol on drop seizures in the lennox-gastaut syndrome
N. Engl. J. Med., 378 (20) (2018), pp. 1888-1897
Crossref View in Scopus Google Scholar
Devinsky et al., 2018b
O. Devinsky, et al.
Open-label use of highly purified CBD (Epidiolex(R)) in patients with CDKL5 deficiency disorder and Aicardi, Dup15q, and Doose syndromes
Epilepsy Behav., 86 (2018), pp. 131-137
View PDF View article View in Scopus Google Scholar
Dubrow et al., 2021
G.A. Dubrow, et al.
A survey of cannabinoids and toxic elements in hemp-derived products from the United States marketplace
J. Food Compos. Anal., 97 (2021), Article 103800
View PDF View article View in Scopus Google Scholar
Ewing et al., 2019a
L.E. Ewing, et al.
Hepatotoxicity of a cannabidiol-rich cannabis extract in the mouse model
Molecules, 24 (9) (2019)
Google Scholar
Ewing et al., 2019b
L.E. Ewing, et al.
Paradoxical patterns of sinusoidal obstruction syndrome-like liver injury in aged female CD-1 mice triggered by cannabidiol-rich cannabis extract and acetaminophen Co-administration
Molecules, 24 (12) (2019)
Google Scholar
FDA, 2018a
FDA
Clinical pharmacology and biopharmaceutical reviews
24 February 2021]; Available from:
https://www.accessdata.fda.gov/drugsatfda_docs/nda/2018/210365Orig1s000ClinPharmR.pdf (2018)
Google Scholar
FDA, 2018b
FDA
Cannabidiol, Epidiolex
24 February 2021]; Available from:
https://www.accessdata.fda.gov/drugsatfda_docs/nda/2018/210365Orig1s000PharmR.pdf (2018)
Google Scholar
FDA, 2018c
FDA
Epidiolex (cannabidiol) oral solution label
30 November 2022]; Available from: https://www.accessdata.fda.gov/drugsatfda_docs/label/2018/210365lbl.pdf (2018)
Google Scholar
FDA, 2018d
FDA
Combined Clinical and statistical review
22 March 2022]; Available from:
https://www.accessdata.fda.gov/drugsatfda_docs/nda/2018/210365Orig1s000MedR.pdf (2018)
Google Scholar
Feinshtein et al., 2013
V. Feinshtein, et al.
Cannabidiol enhances xenobiotic permeability through the human placental barrier by direct inhibition of breast cancer resistance protein: an ex vivo study
Am. J. Obstet. Gynecol., 209 (6) (2013), pp. 573 e1-e573 e15
Google Scholar
Galaj and Xi, 2020
E. Galaj, Z.X. Xi
Possible receptor mechanisms underlying cannabidiol effects on addictive-like behaviors in experimental animals
Int. J. Mol. Sci., 22 (1) (2020)
Google Scholar
Gerard et al., 1990
C. Gerard, et al.
Nucleotide sequence of a human cannabinoid receptor cDNA
Nucleic Acids Res., 18 (23) (1990), p. 7142
Crossref View in Scopus Google Scholar
Gorzalka et al., 2010
B.B. Gorzalka, M.N. Hill, S.C. Chang
Male-female differences in the effects of cannabinoids on sexual behavior and gonadal hormone function
Horm. Behav., 58 (1) (2010), pp. 91-99
View PDF View article View in Scopus Google Scholar
Gulck and Moller, 2020
T. Gulck, B.L. Moller
Phytocannabinoids: origins and biosynthesis
Trends Plant Sci., 25 (10) (2020), pp. 985-1004
View PDF View article View in Scopus Google Scholar
Gustafsson and Jacobsson, 2019
S.B. Gustafsson, S.O.P. Jacobsson
Effects of cannabinoids on the development of chick embryos in ovo
Sci. Rep., 9 (1) (2019), Article 13486
View in Scopus Google Scholar
Harvey and Brown, 1991
D.J. Harvey, N.K. Brown
Comparative in vitro metabolism of the cannabinoids
Pharmacol. Biochem. Behav., 40 (3) (1991), pp. 533-540
View PDF View article View in Scopus Google Scholar
Harvey and Mechoulam, 1990
D.J. Harvey, R. Mechoulam
Metabolites of cannabidiol identified in human urine
Xenobiotica, 20 (3) (1990), pp. 303-320
Crossref View in Scopus Google Scholar
Harvey et al., 1991
D.J. Harvey, E. Samara, R. Mechoulam
Comparative metabolism of cannabidiol in dog, rat and man
Pharmacol. Biochem. Behav., 40 (3) (1991), pp. 523-532
View PDF View article View in Scopus Google Scholar
Hayakawa et al., 2010
K. Hayakawa, K. Mishima, M. Fujiwara
Therapeutic potential of non-psychotropic cannabidiol in ischemic stroke
Pharmaceuticals, 3 (7) (2010), pp. 2197-2212
Crossref View in Scopus Google Scholar
Iffland and Grotenhermen, 2017
K. Iffland, F. Grotenhermen
An update on safety and side effects of cannabidiol: a review of clinical data and relevant animal studies
Cannabis Cannabinoid. Res., 2 (1) (2017), pp. 139-154
Crossref View in Scopus Google Scholar
Jakubovic et al., 1979
A. Jakubovic, E.G. McGeer, P.L. McGeer
Effects of cannabinoids on testosterone and protein synthesis in rat testis Leydig cells in vitro
Mol. Cell. Endocrinol., 15 (1) (1979), pp. 41-50
View PDF View article View in Scopus Google Scholar
Jiang et al., 2011
R. Jiang, et al.
Identification of cytochrome P450 enzymes responsible for metabolism of cannabidiol by human liver microsomes
Life Sci., 89 (5–6) (2011), pp. 165-170
View PDF View article View in Scopus Google Scholar
Laprairie et al., 2015
R.B. Laprairie, et al.
Cannabidiol is a negative allosteric modulator of the cannabinoid CB1 receptor
Br. J. Pharmacol., 172 (20) (2015), pp. 4790-4805
Crossref View in Scopus Google Scholar
Lee et al., 2008
C.Y. Lee, et al.
A comparative study on cannabidiol-induced apoptosis in murine thymocytes and EL-4 thymoma cells
Int. Immunopharm., 8 (5) (2008), pp. 732-740
View PDF View article View in Scopus Google Scholar
Leite et al., 1982
J.R. Leite, et al.
Anticonvulsant effects of the (-) and (+)isomers of cannabidiol and their dimethylheptyl homologs
Pharmacology, 24 (3) (1982), pp. 141-146
View in Scopus Google Scholar
Li et al., 2022
Y. Li, et al.
In vitro effects of cannabidiol and its main metabolites in mouse and human Sertoli cells
Food Chem. Toxicol., 159 (2022), Article 112722
View PDF View article View in Scopus Google Scholar
Martinez et al., 2016
N. Martinez, et al.
TPRV-1 expression in human preeclamptic placenta
Placenta, 40 (2016), pp. 25-28
View PDF View article View in Scopus Google Scholar
Matsuda et al., 1990
L.A. Matsuda, et al.
Structure of a cannabinoid receptor and functional expression of the cloned cDNA
Nature, 346 (6284) (1990), pp. 561-564
View in Scopus Google Scholar
Matsuyama and Fu, 1981
S.S. Matsuyama, T.K. Fu
In vivo cytogenetic effects of cannabinoids
J. Clin. Psychopharmacol., 1 (3) (1981), pp. 135-140
Crossref View in Scopus Google Scholar
McKallip et al., 2006
R.J. McKallip, et al.
Cannabidiol-induced apoptosis in human leukemia cells: a novel role of cannabidiol in the regulation of p22phox and Nox4 expression
Mol. Pharmacol., 70 (3) (2006), pp. 897-908
Crossref View in Scopus Google Scholar
McPartland et al., 2015
J.M. McPartland, et al.
Are cannabidiol and Delta(9) -tetrahydrocannabivarin negative modulators of the endocannabinoid system? A systematic review
Br. J. Pharmacol., 172 (3) (2015), pp. 737-753
Crossref View in Scopus Google Scholar
Mechoulam et al., 2002
R. Mechoulam, L.A. Parker, R. Gallily
Cannabidiol: an overview of some pharmacological aspects
J. Clin. Pharmacol., 42 (S1) (2002), pp. 11S-19
SGoogle Scholar
Millar et al., 2018
S.A. Millar, et al.
A systematic review on the pharmacokinetics of cannabidiol in humans
Front. Pharmacol., 9 (2018), p. 1365
View in Scopus Google Scholar
Millar et al., 2020
S.A. Millar, et al.
Towards better delivery of cannabidiol (CBD)
Pharmaceuticals, 13 (9) (2020)
Google Scholar
Morales et al., 2017
P. Morales, P.H. Reggio, N. Jagerovic
an overview on medicinal chemistry of synthetic and natural derivatives of cannabidiol
Front. Pharmacol., 8 (2017), p. 422
View in Scopus Google Scholar
Munro et al., 1993
S. Munro, K.L. Thomas, M. Abu-Shaar
Molecular characterization of a peripheral receptor for cannabinoids
Nature, 365 (6441) (1993), pp. 61-65
View in Scopus Google Scholar
Narimatsu et al., 1988
S. Narimatsu, et al.
Mechanism for inhibitory effect of cannabidiol on microsomal testosterone oxidation in male rat liver
Drug Metab. Dispos., 16 (6) (1988), pp. 880-889
View in Scopus Google Scholar
Neradugomma et al., 2019
N.K. Neradugomma, et al.
Marijuana-derived cannabinoids inhibit uterine endometrial stromal cell decidualization and compromise trophoblast-endometrium cross-talk
Reprod. Toxicol., 87 (2019), pp. 100-107
View PDFView article View in Scopus Google Scholar
Nouri et al., 2021
K. Nouri, et al.
Involvement of hippocampal D1-like dopamine receptors in the inhibitory effect of cannabidiol on acquisition and expression of methamphetamine-induced conditioned place preference
Neurochem. Res., 46 (8) (2021), pp. 2008-2018
Crossref View in Scopus Google Scholar
Ou et al., 2020
O. Ou, et al.
Cannabidiol (CBD)-Related adverse events reports from the FDA CFSAN adverse event reporting system (CAERS)
2021 FDA Science Forum (2020)
https://www.fda.gov/science-research/fda-science-forum/cannabidiol-cbd-related-adverse-events-reports-fda-cfsan-adverse-event-reporting-system-caers-2020
Google Scholar
Paton and Pertwee, 1972
W.D. Paton, R.G. Pertwee
Effect of cannabis and certain of its constituents on pentobarbitone sleeping time and phenazone metabolism
Br. J. Pharmacol., 44 (2) (1972), pp. 250-261
Crossref View in Scopus Google Scholar
Pennypacker and Romero-Sandoval, 2020
S.D. Pennypacker, E.A. Romero-Sandoval
CBD and THC: do they complement each other like yin and yang
Pharmacotherapy, 40 (11) (2020), pp. 1152-1165
Crossref View in Scopus Google Scholar
Pertwee et al., 2010
R.G. Pertwee, et al.
International union of basic and clinical pharmacology. LXXIX. Cannabinoid receptors and their ligands: beyond CB(1) and CB(2)
Pharmacol. Rev., 62 (4) (2010), pp. 588-631
Crossref View in Scopus Google Scholar
Perucca and Bialer, 2020
E. Perucca, M. Bialer
Critical aspects affecting cannabidiol oral bioavailability and metabolic elimination, and related clinical implications
CNS Drugs, 34 (8) (2020), pp. 795-800
Crossref View in Scopus Google Scholar
Ren et al., 2021
G. Ren, et al.
Large-scale whole-genome resequencing unravels the domestication history of Cannabis sativa
Sci. Adv., 7 (29) (2021)
Google Scholar
Rosenkrantz et al., 1981
H. Rosenkrantz, R.W. Fleischman, R.J. Grant
Toxicity of short-term administration of cannabinoids to rhesus monkeys
Toxicol. Appl. Pharmacol., 58 (1) (1981), pp. 118-131
View PDF View article View in Scopus Google Scholar
Russo et al., 2019
C. Russo, et al.
Low doses of widely consumed cannabinoids (cannabidiol and cannabidivarin) cause DNA damage and chromosomal aberrations in human-derived cells
Arch. Toxicol., 93 (1) (2019), pp. 179-188
Crossref View in Scopus Google Scholar
Schuel et al., 1991
H. Schuel, et al.
Cannabinoids inhibit fertilization in sea urchins by reducing the fertilizing capacity of sperm
Pharmacol. Biochem. Behav., 40 (3) (1991), pp. 609-615
View PDF View article View in Scopus Google Scholar
Shahbazi et al., 2020
F. Shahbazi, et al.
Cannabinoids and cannabinoid receptors: the story so far
iScience, 23 (7) (2020), Article 101301
View PDF View article View in Scopus Google Scholar
Silver, 2019
R.J. Silver
The endocannabinoid system of animals
Animals (Basel), 9 (9) (2019)
Google Scholar
Soethoudt et al., 2017
M. Soethoudt, et al.
Cannabinoid CB2 receptor ligand profiling reveals biased signalling and off-target activity
Nat. Commun., 8 (2017), Article 13958
View in Scopus Google Scholar
Sugiura and Waku, 2002
T. Sugiura, K. Waku
Cannabinoid receptors and their endogenous ligands
J. Biochem., 132 (1) (2002), pp. 7-12
Crossref View in Scopus Google Scholar
Thiele et al., 2018
E.A. Thiele, et al.
Cannabidiol in patients with seizures associated with Lennox-Gastaut syndrome (GWPCARE4): a randomised, double-blind, placebo-controlled phase 3 trial
Lancet, 391 (10125) (2018), pp. 1085-1096
View PDF View article View in Scopus Google Scholar
Thomas et al., 2007
A. Thomas, et al.
Cannabidiol displays unexpectedly high potency as an antagonist of CB1 and CB2 receptor agonists in vitro
Br. J. Pharmacol., 150 (5) (2007), pp. 613-623
Crossref View in Scopus Google Scholar
Uhlen et al., 2015
M. Uhlen, et al.
Proteomics. Tissue-based map of the human proteome
Science, 347 (6220) (2015), Article 1260419
View in Scopus Google Scholar
Ujvary and Hanus, 2016
I. Ujvary, L. Hanus
Human metabolites of cannabidiol: a review on their formation, biological activity, and relevance in therapy
Cannabis Cannabinoid. Res., 1 (1) (2016), pp. 90-101
Crossref View in Scopus Google Scholar
USDA, 2019
USDA
Executive summary of new hemp authorities
25 January 2022]; Available from:
https://www.ams.usda.gov/sites/default/files/HempExecSumandLegalOpinion.pdf (2019)
Google Scholar
van den Elsen et al., 2014
G.A. van den Elsen, et al.
Efficacy and safety of medical cannabinoids in older subjects: a systematic review
Ageing Res. Rev., 14 (2014), pp. 56-64
View PDF View article View in Scopus Google Scholar
Wallace et al., 2015
M.S. Wallace, et al.
Efficacy of inhaled cannabis on painful diabetic neuropathy
J. Pain, 16 (7) (2015), pp. 616-627
View PDF View article View in Scopus Google Scholar
Wanner et al., 2021
N.M. Wanner, et al.
Developmental cannabidiol exposure increases anxiety and modifies genome-wide brain DNA methylation in adult female mice
Clin. Epigenet., 13 (1) (2021), p. 4
View in Scopus Google Scholar
Watanabe et al., 2005
K. Watanabe, et al.
Marijuana extracts possess the effects like the endocrine disrupting chemicals
Toxicology, 206 (3) (2005), pp. 471-478
View PDF View article View in Scopus Google Scholar
Watkins et al., 2021
P.B. Watkins, et al.
Cannabidiol and abnormal liver chemistries in healthy adults: results of a phase I clinical trial
Clin. Pharmacol. Ther., 109 (5) (2021), pp. 1224-1231
Crossref View in Scopus Google Scholar
WHO, 2018
WHO
Cannabidiol (CBD): critical review report
Exp. Comm. Drug Depend. (2018)
24 February 2021]; Available from:
https://www.who.int/medicines/access/controlled-substances/CannabidiolCriticalReview.pdf
Google Scholar
Wu et al., 2008
H.Y. Wu, et al.
Cannabidiol-induced apoptosis in primary lymphocytes is associated with oxidative stress-dependent activation of caspase-8
Toxicol. Appl. Pharmacol., 226 (3) (2008), pp. 260-270
View PDF View article View in Scopus Google Scholar
Xiong et al., 2011
W. Xiong, et al.
Cannabinoid potentiation of glycine receptors contributes to cannabis-induced analgesia
Nat. Chem. Biol., 7 (5) (2011), pp. 296-303
Crossref View in Scopus Google Scholar
Yamamoto et al., 1995
I. Yamamoto, et al.
Recent advances in the metabolism of cannabinoids
Int. J. Biochem. Cell Biol., 27 (8) (1995), pp. 741-746
View PDF View article View in Scopus Google Scholar
Zimmerman and Raj, 1980
A.M. Zimmerman, A.Y. Raj
Influence of cannabinoids on somatic cells in vivo
Pharmacology, 21 (4) (1980), pp. 277-287
Crossref View in Scopus Google Scholar
Zimmerman and Zimmerman, 1990
S. Zimmerman, A.M. Zimmerman
Genetic effects of marijuana
Int. J. Addict., 25 (1A) (1990), pp. 19-33
Crossref View in Scopus Google Scholar
Zuardi et al., 2006
A.W. Zuardi, et al.
Cannabidiol, a Cannabis sativa constituent, as an antipsychotic drug
Braz. J. Med. Biol. Res., 39 (4) (2006), pp. 421-429
View in Scopus Google Scholar