機能的な SARS-CoV-2 特異的免疫記憶は軽度の COVID-19 後も持続(2020年8月15日)
元→Microsoft Word - Pepper Ms Nature 200810 for PDF.docx (medrxiv.org)
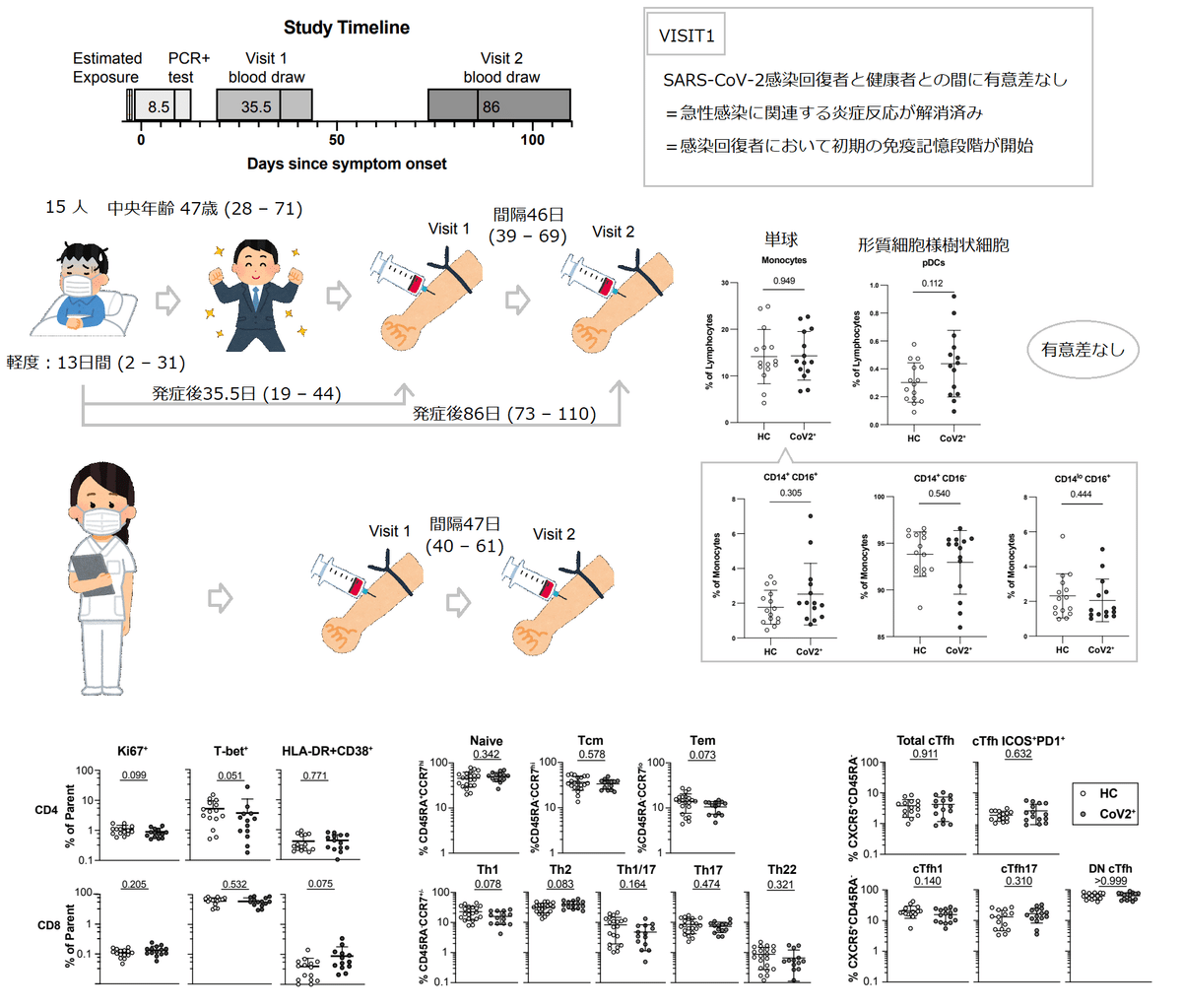
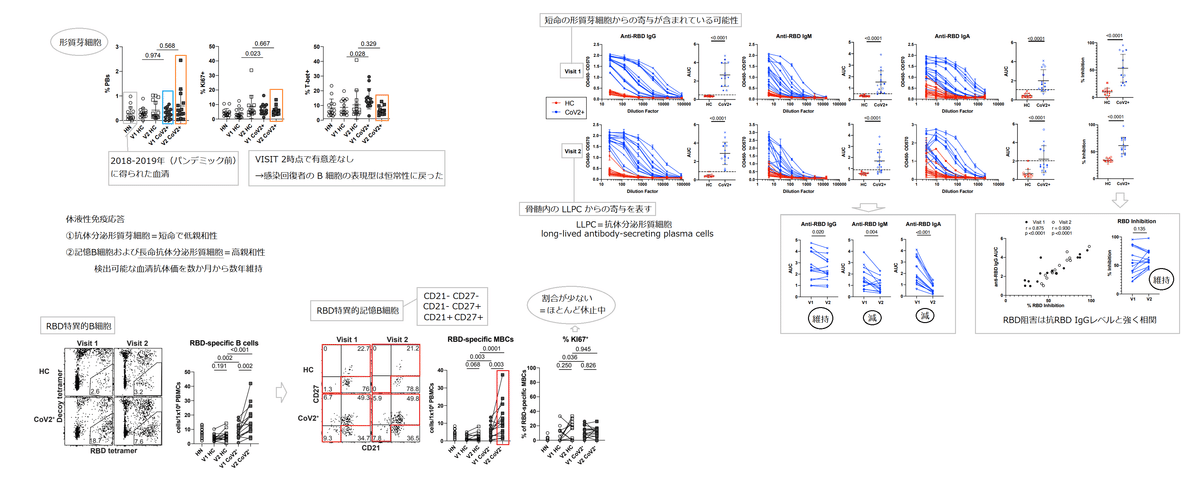
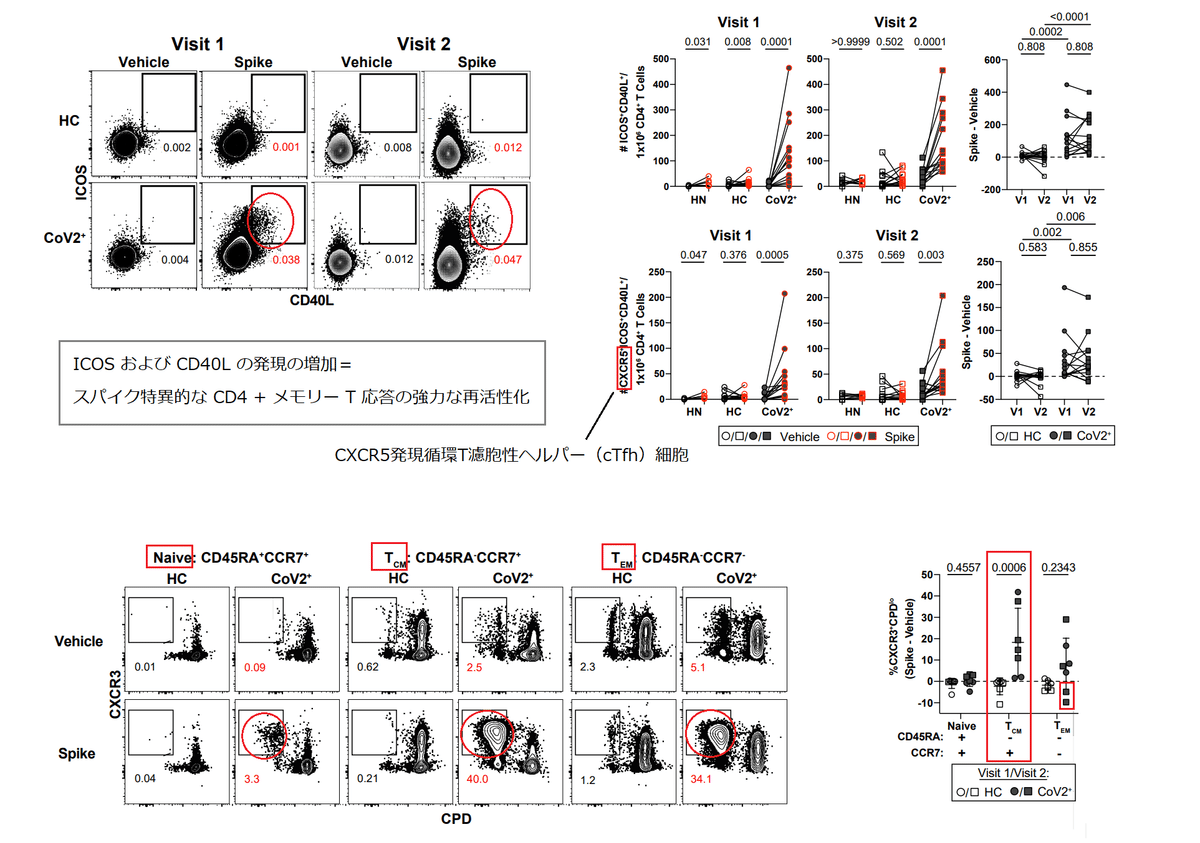
Functional SARS-CoV-2-specific immune memory persists after mild COVID-19
機能的な SARS-CoV-2 特異的免疫記憶は軽度の COVID-19 後も持続する
Summary:
The recently emerged SARS-CoV-2 virus is currently causing a global pandemic and cases continue to rise.
最近出現した SARS-CoV-2 ウイルスは現在、世界的な大流行を引き起こし、症例は増加し続けています。
The majority of infected individuals experience mildly symptomatic coronavirus disease 2019 (COVID-19), but it is unknown whether this can induce persistent immune memory that might contribute to herd immunity.
感染者の大部分は、軽度の症候性コロナウイルス病 2019 (COVID-19) を経験しますが、これが集団免疫に寄与する持続的な免疫記憶を誘発できるかどうかは不明です。
Thus, we performed a longitudinal assessment of individuals recovered from mildly symptomatic COVID-19 to determine if they develop and sustain immunological memory against the virus.
したがって、軽度の症状のCOVID-19から回復した個人の縦断的評価を実施して、ウイルスに対する免疫記憶を発達させ、維持しているかどうかを判断しました。
We found that recovered individuals developed SARS-CoV-2-specific IgG antibody and neutralizing plasma, as well as virus-specific memory B and T cells that not only persisted, but in some cases increased numerically over three months following symptom onset.
回復した個人は、SARS-CoV-2 特異的 IgG 抗体と中和血漿を発達させることがわかりました。ウイルス特異的なメモリー B および T 細胞は、持続するだけでなく、場合によっては、症状の発症後 3 か月にわたって数値的に増加しました。
Furthermore, the SARS-CoV-2-specific memory lymphocytes exhibited characteristics associated with potent antiviral immunity: memory T cells secreted IFN-γ and expanded upon antigen re-encounter, while memory B cells expressed receptors capable of neutralizing virus when expressed as antibodies.
さらに、SARS-CoV-2 特異的記憶リンパ球は、強力な抗ウイルス免疫に関連する特徴を示しました:メモリー T 細胞は IFN-γ を分泌し、抗原との再遭遇時に増殖します。一方、記憶B細胞は、抗体として発現するとウイルスを中和できる受容体を発現しました。
These findings demonstrate that mild COVID-19 elicits memory lymphocytes that persist and display functional hallmarks associated with antiviral protective immunity.
これらの調査結果は、軽度の COVID-19 が記憶リンパ球を誘発し、抗ウイルス防御免疫に関連する機能的特徴を持続して示すことを示しています。
Main Text:
The rapid spread of the SARS-CoV-2 beta coronavirus has infected 19 million and killed over 700,000 people worldwide as of early August 2020.
SARS-CoV-2 ベータ コロナウイルスの急速な拡散により、2020 年 8 月初旬の時点で、世界中で 1,900 万人が感染し、70 万人以上が死亡しています。
Infection causes the disease COVID-19, which ranges in presentation from asymptomatic to fatal.
感染症は COVID-19 という病気を引き起こしますが、その症状は無症候性から致命的なものまでさまざまです。
However, the vast majority of infected individuals experience mild symptoms that do not require hospitalization.
ただし、感染者の大多数は、入院を必要としない軽度の症状を経験します。
It is critically important to understand if SARS-CoV-2–infected individuals who recover from mild disease develop immune memory that protects them from subsequent SARS-CoV-2 infections, thereby reducing transmission and promoting herd immunity.
軽度の疾患から回復した SARS-CoV-2 感染者が、その後の SARS-CoV-2 感染から保護する免疫記憶を発達させるかどうかを理解することは非常に重要です。 それにより、伝染を減らし、集団免疫を促進します。
Immunological memory is predominantly mediated by cells of the adaptive immune system.
免疫記憶は、主に適応免疫系の細胞によって媒介されます。
In response to most acute viral infections, B and T cells that can bind viral antigens through their antigen receptors become activated, expand, differentiate and begin secreting effector molecules to help control the infection.
ほとんどの急性ウイルス感染に応答して、抗原受容体を介してウイルス抗原に結合できる B 細胞および T 細胞が活性化され、拡大し、分化し、エフェクター分子を分泌して感染の制御を助けます。
Upon resolution of infection, approximately 90% of these virus-specific “effector cells” die, while 10% persist as long-lived “memory” cells.
感染が解消すると、これらのウイルス特異的な「エフェクター細胞」の約 90% が死滅しますが、10% は長命の「記憶」細胞として存続します。
Immune memory cells can produce a continuous supply of effector molecules, as seen with long-lived antibody-secreting plasma cells (LLPCs).
免疫記憶細胞は、長寿命の抗体分泌形質細胞 (LLPC) で見られるように、エフェクター分子の継続的な供給を生成できます。
In most cases, however, quiescent memory lymphocytes are strategically positioned to rapidly reactivate in response to re-infection and execute effector programs imprinted upon them during the primary response.
ただし、ほとんどの場合、静止記憶リンパ球は、再感染に反応して急速に再活性化し、一次反応中にそれらに刻印されたエフェクタープログラムを実行するように戦略的に配置されています。
Upon re-infection, pathogen-specific memory B cells (MBCs) that express receptors associated with antigen experience and the transcription factor T-bet rapidly proliferate and differentiate into IgG+ antibody-secreting plasmablasts (PBs).
再感染すると、抗原経験と転写因子 T-bet に関連する受容体を発現する病原体特異的記憶 B 細胞 (MBC) が急速に増殖し、IgG+ 抗体分泌形質芽細胞 (PB) に分化します。
Reactivated T-bet–expressing memory CD4+ T cells proliferate, “help” activate MBCs and secrete cytokines (including IFNγ) to activate innate cells.
再活性化された T-bet 発現メモリー CD4+ T 細胞は増殖し、MBC の活性化を「助け」、自然細胞を活性化するためにサイトカイン (IFNγ を含む) を分泌します。
Meanwhile, memory CD8+T cells can kill virus-infected cells directly through the delivery of cytolytic molecules.
一方、メモリーCD8+T細胞は、細胞溶解分子の送達を通じてウイルス感染細胞を直接殺すことができます。
These quantitatively and qualitatively enhanced virus-specific memory populations coordinate to quickly clear the virus, thereby preventing disease and reducing the chance of transmission.
これらの量的および質的に強化されたウイルス固有の記憶集団は、ウイルスを迅速に排除するために協調します。 これにより、病気を予防し、感染の可能性を減らします。
To infect cells and propagate, SARS-CoV-2 relies on the interaction between the receptor binding domain (RBD) of its spike protein (S) and angiotensin converting enzyme 2 (ACE2) on host cells.
細胞に感染して増殖するために、SARS-CoV-2 は、そのスパイクタンパク質 (S) の受容体結合ドメイン (RBD) と宿主細胞上のアンギオテンシン変換酵素 2 (ACE2) との間の相互作用に依存しています。
Multiple studies have shown that the majority of SARS-CoV-2 infected individuals produce S- and RBD-specific antibodies during the primary response, and RBD-specific monoclonal antibodies can neutralize the virus in vitro and in vivo.
複数の研究により、SARS-CoV-2 感染者の大部分が一次応答中に S および RBD 特異的抗体を産生し、RBD 特異的モノクローナル抗体が in vitro および in vivo でウイルスを中和できることが示されています。
Therefore, RBD-specific antibodies would likely contribute to protection against re-infection if expressed by LLPCs or MBCs.
したがって、RBD 特異的抗体は、LLPC または MBC によって発現された場合、再感染に対する保護に寄与する可能性があります。
To determine if the above hallmarks of immune protection from viral infection both form and persist in individuals that have experienced mild COVID-19, we assessed their SARS-CoV-2-specific immune responses at one and three months post-symptom onset.
ウイルス感染からの免疫保護の上記の特徴が、軽度の COVID-19 を経験した個人で形成され持続するかどうかを判断するために、発症後 1 か月および 3 か月での SARS-CoV-2 特異的免疫応答を評価しました。
Herein we demonstrate that a multipotent SARS-CoV-2-specific immune memory response forms and is maintained in recovered individuals at least for the duration of our study.
ここで、多能性のSARS-CoV-2特異的免疫記憶応答が形成され、少なくとも研究期間中は回復した個人で維持されることを示しています。
Furthermore, memory lymphocytes display hallmarks of protective antiviral immunity.
さらに、記憶リンパ球は防御抗ウイルス免疫の特徴を示します。
Return to immune homeostasis after mildly symptomatic COVID-19
軽度の症候性COVID-19の後、免疫恒常性に戻る
To determine if immune memory cells form after mildly symptomatic COVID-19, we collected plasma and peripheral blood mononuclear cells (PBMCs) from 15 individuals recovered from COVID-19 (CoV2+) (UW IRB 00009810).
症状が軽度の COVID-19 の後に免疫記憶細胞が形成されるかどうかを判断するために、COVID-19 (CoV2+) から回復した 15 人の個人から血漿および末梢血単核細胞 (PBMC) を収集しました (UW IRB 00009810)。
The CoV2+ group had a median age of 47 and reported mild symptoms lasting a median of 13 days (E.D. Table 1).
CoV2+ グループの年齢の中央値は 47 歳で、軽度の症状が中央値で 13 日間続いたと報告されています (E.D. 表 1)。
The first blood sample (Visit 1) was drawn at least 20 days after a positive PCR test for SARS-CoV-2 and a median of 35.5 days post-symptom onset.
最初の血液サンプル (Visit 1) は、SARS-CoV-2 の PCR 検査陽性の少なくとも 20 日後に採取され、症状発症後中央値は 35.5 日でした。
We expect the primary response to be contracting and early memory populations to be generated at this time point, as viral load is cleared approximately 8 days post symptom onset.
症状の発症から約8日後にウイルス負荷が解消されるため、この時点で一次反応は収縮し、初期の記憶集団が生成されると予想されます。
Participants returned for a second blood draw (Visit 2) a median of 86 days post-symptom onset so we could assess the quantity and quality of the long-lived memory populations (Fig. 1a).
参加者は、発症後86日の中央値で2回目の採血(訪問2)のために戻ってきたので、長寿命の記憶集団の量と質を評価できました(図1a)。
We compared these samples to samples collected at two time points representing a similar sampling interval in a group of 17 healthy controls (HCs).
これらのサンプルを、17 人の健康なコントロール (HC) のグループで同様のサンプリング間隔を表す 2 つの時点で収集されたサンプルと比較しました。
All HCs were considered to have no prior SARS-CoV-2 infection based on having no detectable plasma SARS-CoV-2 RBD- or S-specific antibodies above three standard deviations (SDs) of the mean of historical negative (HN) plasma samples (E.D. Fig. 1).
すべてのHCは、過去の陰性(HN)血漿サンプルの平均の3標準偏差(SD)を超える検出可能な血漿SARS-CoV-2 RBDまたはS特異的抗体を持たないことに基づいて、以前にSARS-CoV-2に感染していないと見なされました (ED図1)。
We also included HN PBMC samples that were collected prior to the first human SARS-CoV-2 infection (2016-2019).
また、最初のヒト SARS-CoV-2 感染 (2016-2019) の前に収集された HN PBMC サンプルも含めました。
We included these to control for the possibility that individuals in the HC group had been infected with SARS-CoV-2 (9/17 described having some symptoms associated with SARS-CoV-2 infection) despite their lack of detectable RBD-specific antibodies.
検出可能なRBD特異的抗体はないですが、HCグループの個人がSARS-CoV-2に感染した可能性を制御するためにこれらを含めました(9/17はSARS-CoV-2感染に関連するいくつかの症状があると説明しました)。
Populations of activated innate and adaptive immune cells expand in the blood during the primary response to SARS-CoV-2 infection.
活性化された自然免疫および適応免疫細胞の集団は、SARS-CoV-2 感染に対する一次応答中に血液中で拡大します。
When an acute viral infection is cleared, the majority of these highly inflammatory cells either die or become quiescent memory cells such that the proportions and phenotypes of total immune cells are indistinguishable from those seen in pre-infection blood samples.
急性ウイルス感染が解消されると、これらの高度に炎症を起こした細胞の大部分が死ぬか、静止記憶細胞になるため、総免疫細胞の割合と表現型は、感染前の血液サンプルで見られるものと区別できなくなります。
Consistent with resolution of the primary response, we found no differences in frequency of total monocytes, monocyte subsets or plasmacytoid dendritic cells among PBMCs between CoV2+ and HC individuals (E.D. Fig. 2).
一次応答の解像度と一致して、CoV2+ と HC の個人間の PBMC 間で、総単球、単球サブセット、または形質細胞様樹状細胞の頻度に違いは見られませんでした (E.D. 図 2)。
We also found no differences in γδ or αβ CD3+ T cell frequencies (CD4+ or CD8+), nor in the cell cycle status, expression of molecules associated with activation, migration, function or proportions of various CD45RA – memory T cell subsets (E.D. Fig. 3).
また、γδ または αβ CD3+ T 細胞の頻度 (CD4+ または CD8+) にも、細胞周期の状態にも、さまざまな CD45RA – メモリー T 細胞サブセットの活性化、移動、機能、または比率に関連する分子の発現にも違いは見られませんでした(ED図3)。
Together, these data demonstrate that the inflammatory response associated with acute infection had resolved by the Visit 1 time point and the early immune memory phase had commenced.
まとめると、これらのデータは、急性感染に関連する炎症反応が訪問 1 の時点までに解消され、初期の免疫記憶段階が開始したことを示しています。
Mild COVID-19 induces persistent, neutralizing anti-SARS-CoV-2 IgG antibody
軽度の COVID-19 は持続性の中和抗 SARS-CoV-2 IgG 抗体を誘導する
Humoral immune responses are characterized by a first wave of short-lived, low-affinity antibody-secreting PBs followed by a subsequent germinal center (GC) response that generates high-affinity MBCs and antibody-secreting LLPCs.
体液性免疫応答は、短命で低親和性の抗体分泌 PB の最初の波と、それに続く高親和性 MBC および抗体分泌 LLPC を生成する胚中心 (GC) 応答によって特徴付けられます。
LLPCs can maintain detectable serum antibody titers for months to many years, depending upon the specific viral infection.
LLPC は、特定のウイルス感染に応じて、検出可能な血清抗体価を数か月から数年維持できます。
Thus, it is critical to distinguish the first wave of waning PB-derived antibodies from the later wave of persistent LLPC-derived antibodies that can neutralize subsequent infections, potentially for life.
したがって、PB 由来の抗体が減少する最初の波と、その後の感染を潜在的に生涯中和できる持続的な LLPC 由来の抗体の後の波を区別することが重要です。
We therefore first determined that CD19+CD20loCD38hi PBs were no longer present at elevated frequencies in CoV2+ individuals relative to HCs at Visit 1 (E.D. Fig. 4a).
したがって、CD19 + CD20lo CD38hi PBは、VISIT 1 でHCと比較してCoV2 +個体に高い頻度で存在しなくなったことを最初に確認しました(E.D.図4a)。
Other measures of recent B cell activation in non-PB B cells include increased Ki67 expression (indicating cells have entered the cell cycle) and expression of T-bet.
非 PB B 細胞における最近の B 細胞活性化の他の尺度には、Ki67 発現の増加 (細胞が細胞周期に入ったことを示す) および T-bet の発現が含まれます。
There are small increases in both the frequencies of Ki67+ and T-bet+ B cells at the Visit 1 time point compared to HC, but not at the Visit 2 time point (E.D. Fig. 4b,c).
HC と比較してVISIT 1 の時点で Ki67+ および T-bet+ B 細胞の頻度の両方にわずかな増加がありますが、VISIT 2 の時点では増加していません (E.D. 図 4b、c)。
These data suggest that while PBs associated with controlling acute infection are no longer detectable in CoV2+ individuals at Visit 1, other B cell fates are still contracting.
これらのデータは、急性感染の制御に関連する PB は、VISIT 1 で CoV2+ 患者ではもはや検出されていませんが、他の B 細胞運命はまだ縮小していることを示唆しています。
However, by Visit 2, these B cell phenotypes have returned to homeostasis (E.D. Fig. 4a-c).
しかし、訪問 2 までに、これらの B 細胞の表現型は恒常性に戻りました (E.D. 図 4a-c)。
Antibodies measured at Visit 1 might include contributions from short-lived plasmablasts, while those measured at Visit 2, long after PBs have contracted, represent contributions from LLPCs in the bone marrow.
Visit 1 で測定された抗体には短命の形質芽細胞からの寄与が含まれている可能性がありますが、Visit 2 で測定された抗体は、PB が収縮してからかなり後に測定され、骨髄内の LLPC からの寄与を表しています。
We therefore examined the SARS-CoV-2-specific IgG, IgM and IgA antibodies at Visit 1 and Visit 2.
したがって、Visit1とVisit2でSARS-CoV-2特異的IgG、IgM、およびIgA抗体を調べました。
At Visit 1, 100% of CoV2+ individuals had plasma anti-RBD IgG levels 3 SDs above the mean of HCs, as measured by ELISA area under the curve (AUC), in accordance with studies showing 100% seroprevalence by day 14(Fig. 1b).
Visit 1 で、CoV2+ 患者の 100% は、ELISA 曲線下面積 (AUC) で測定して、HC の平均よりも 3 SD 高い血漿抗 RBD IgG レベルを有していました。14日目までに100%の血清有病率を示しています (図1b)。
Additionally, 93% of CoV2+ individuals had anti-RBD IgM and 73% had anti-RBD IgA above this negative threshold.
さらに、CoV2+ 患者の 93% が抗 RBD IgM を有し、73% がこのネガティブの閾値を超える抗 RBD IgA を有していました。
Almost all CoV2+ individuals possessed IgG (100%), IgM (100%), and IgA (93%) anti-spike antibodies above the threshold at Visit 1 as well (E.D. Fig. 4d).
ほとんどすべての CoV2+ 患者は、訪問 1 の時点でも閾値を超える IgG (100%)、IgM (100%)、および IgA (93%) 抗スパイク抗体を保有していました (E.D. 図 4d)。
Levels of anti-RBD and anti-spike binding were highly correlated for all isotypes (E.D. Fig. 4e).
抗RBDおよび抗スパイク結合のレベルは、すべてのアイソタイプで高度に相関していました(ED図4e)。
At Visit 2, all CoV2+ individuals maintained anti-RBD IgG levels above the negative threshold and 71% and 36% had maintained anti-RBD IgM and IgA, respectively (Fig. 1b).
訪問 2 では、すべての CoV2+ 患者が抗 RBD IgG レベルをネガティブの閾値より上に維持し、71% と 36% がそれぞれ抗 RBD IgM と IgA を維持していました (図 1b)。
Anti-RBD IgG levels decreased only slightly among CoV2+ individuals between time points and 36% of CoV2+ individuals had the same or increased levels at Visit 2.
抗RBD IgGレベルは、CoV2 +個人の間で時点間でわずかにしか減少しませんでした。また、CoV2+ 患者の 36% は、訪問 2 で同じか増加したレベルでした。
Anti-RBD IgM and IgA, however, decreased substantially from Visit 1 to Visit 2 (Fig. 1c, E.D. Fig. 4f).
ただし、抗RBD IgMおよびIgAは、訪問1から訪問2にかけて大幅に減少しました(図1c、ED図4f)。
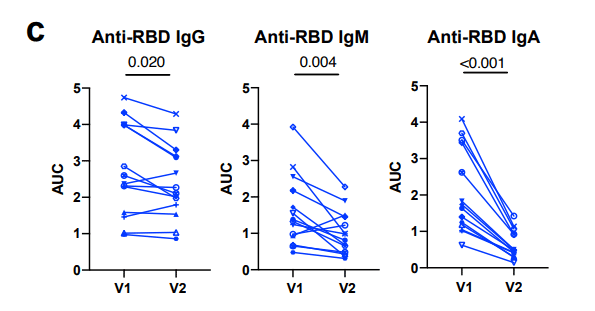
As spike protein, and specifically the RBD, is key for viral entry into the cell, antibodies that target the RBD can be potent inhibitors of infection.
スパイクタンパク質、特に RBD はウイルスが細胞に侵入するための鍵であるため、RBD を標的とする抗体は感染の強力な阻害剤となる可能性があります。
To determine whether CoV2+ individuals form and maintain neutralizing antibodies, we tested for SARS-CoV-2 neutralization indirectly using a cell-free competition assay (surrogate virus neutralization test, sVNT) and directly in a plaque reduction neutralization test (PRNT).
CoV2+ 個体が中和抗体を形成して維持するかどうかを判断するために、無細胞競合アッセイ (代理ウイルス中和試験、sVNT) を使用して間接的に SARS-CoV-2 中和をテストし、プラーク減少中和テスト (PRNT) で直接テストしました。
CoV2+ plasma inhibited RBD binding to ACE2 significantly more than HC plasma by sVNT and RBD inhibition correlated strongly with anti-RBD IgG levels at both time points (Fig. 1d,e).
CoV2 +血漿は、sVNTによってHC血漿よりもACE2へのRBD結合を有意に阻害し、RBD阻害は両方の時点で抗RBD IgGレベルと強く相関しました(図1d、e)。
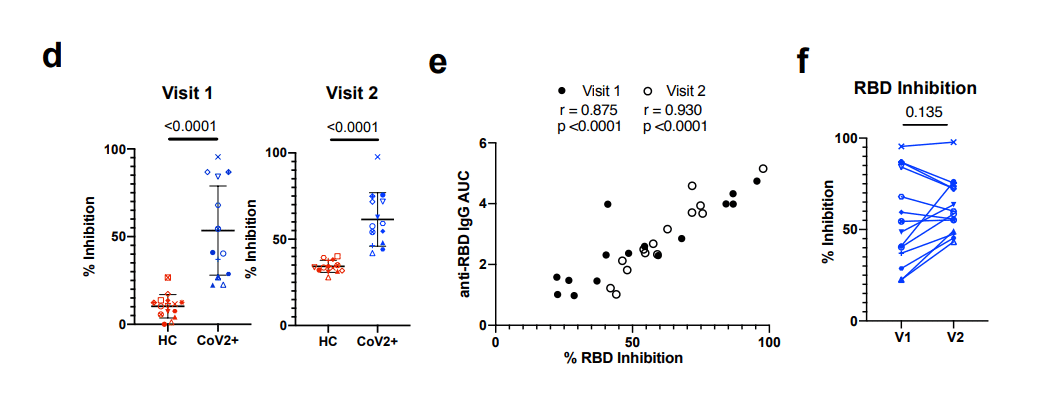
Further, RBD inhibition capacity was maintained or increased in the majority of CoV2+ individuals from Visit 1 to Visit 2 (Fig. 1f, E.D. Fig. 4g).
さらに、Visit 1 からVisit 2 まで、大部分の CoV2+ 患者で RBD 阻害能が維持または増加しました (図 1f、E.D. 図 4g)。
Neutralization by PRNT correlated strongly with RBD inhibition at both time points (Fig. 1g, E.D. 4h) and was similarly maintained between visits (Fig. 1h).
PRNTによる中和は、両方の時点でRBD阻害と強く相関し(図1g、ED 4h)、Visit 間で同様に維持されました(図1h)。
By the latest time point in our study, 86% of CoV2+ individuals still had better RBD-inhibiting plasma than HCs and 71% had better neutralizing plasma (measured as above HC mean + 3 SDs).
私たちの研究の最新の時点までに、CoV2 +個人の86%は依然としてHCよりもRBD阻害血漿が良好であり、71%は中和血漿が良好でした(HC平均+ 3 SDとして測定)。
These data are consistent with the emergence of predominantly IgG+ RBD and spike-specific LLPCs that maintain detectable neutralizing anti-SARS-CoV-2 antibody to at least 3 months post-symptom onset.
これらのデータは、主に IgG+ RBD およびスパイク特異的 LLPC の出現と一致しており、これらは検出可能な中和抗 SARS-CoV-2 抗体を発症後少なくとも 3 か月間維持します。
Mild COVID-19 induces a sustained enrichment of RBD-specific memory B cells
軽度の COVID-19 は、RBD 特異的記憶 B 細胞の持続的な濃縮を誘導します
The presence of SARS-CoV-2-neutralizing antibodies three months post-symptom onset in CoV2+ individuals suggests GC-derived memory LLPCs have formed.
CoV2+ 患者における発症から 3 か月後の SARS-CoV-2 中和抗体の存在は、GC 由来のメモリー LLPC が形成されたことを示唆しています。
GC-derived MBCs also play a critical role in the formation of antibody secreting cells upon antigen re-exposure.
GC 由来の MBC は、抗原の再曝露による抗体分泌細胞の形成にも重要な役割を果たします。
Therefore, we tested whether SARS-CoV-2-specific MBCs were also formed and maintained in CoV2+ individuals throughout the study time course.
したがって、SARS-CoV-2 固有の MBC も形成され、研究の時間経過を通じて CoV2+ 個体で維持されるかどうかをテストしました。
We generated RBD tetramer reagents and used enrichment strategies to identify rare RBD-specific cells that are otherwise undetectable in bulk assessments.
RBD テトラマー試薬を生成し、濃縮戦略を使用して、バルク評価では検出できない希少な RBD 特異的細胞を特定しました。
We confirmed specificity in RBD immunized mice and then used the RBD-tetramer to identify, enumerate and phenotype rare, RBD-specific B cells in our HN, HC and CoV2+ individuals (E.D. Fig. 5a,b; Fig. 2a).
RBD免疫マウスで特異性を確認し、RBDテトラマーを使用して、HN、HC、およびCoV2 +個体のまれなRBD特異的B細胞を識別、列挙、および表現型しました(ED図5a、b;図2a)。
Gates used to phenotype RBD-specific B cells were defined on total B cell populations (E.D. Fig. 5c).
RBD特異的B細胞の表現型に使用されるゲートは、総B細胞集団で定義されました(ED図5c)。
At Visit 1, RBD-specific B cells were significantly expanded in CoV2+ individuals compared to HCs and their numbers were increased further at Visit 2 (Fig. 2a, b).
訪問 1 では、RBD 特異的 B 細胞は HC と比較して CoV2+ 個体で有意に増加し、その数は訪問 2 でさらに増加しました (図 2a、b)。
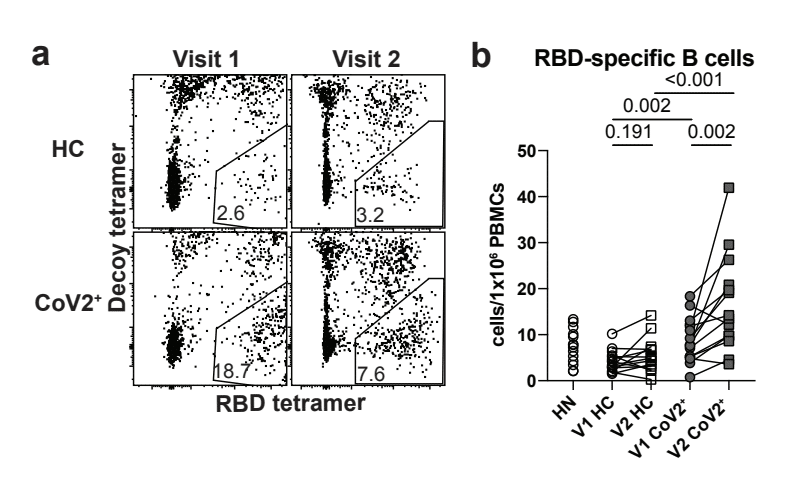
The proportion and number of RBD-specific MBCs (defined by CD21 and CD27 expression) in CoV2+ samples was significantly greater than in HCs and increased from Visit 1 to Visit 2 (Fig. 2c,d, E.D. Fig. 5d).
CoV2+ サンプル中の RBD 特異的 MBC (CD21 および CD27 発現によって定義される) の割合と数は、HC よりも有意に大きく、Visit 1 から Visit 2 にかけて増加しました (図 2c、d、E.D. 図 5d)。
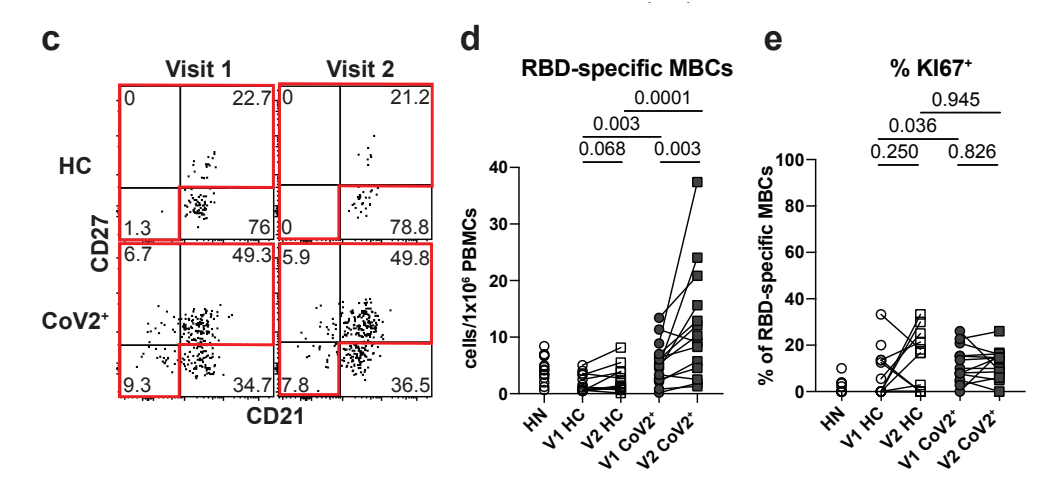
While RBD-specific B cells in HN samples had a similar proportion of MBCs as in CoV2+ samples, they contained substantially fewer cells.
HNサンプルのRBD特異的B細胞は、CoV2+サンプルと同様の割合のMBCを持っていましたが、含まれる細胞は大幅に少なかった。
In addition, RBD-specific MBCs were largely quiescent with very few expressing Ki67.
さらに、RBD 固有の MBC は大部分が静止しており、Ki67 の発現はほとんどありませんでした。
We therefore assayed BCR isotype expression on RBD-specific MBCs and found enriched populations of IgA- and IgG-expressing MBCs in CoV2+ individuals at both time points (Fig. 2f-h, E.D. Fig. 5f).
したがって、RBD固有のMBCでBCRアイソタイプの発現をアッセイしました。両方の時点で、CoV2 +個人でIgAおよびIgG発現MBCの濃縮集団が見つかりました(図2f〜h、E.D.図5f)。
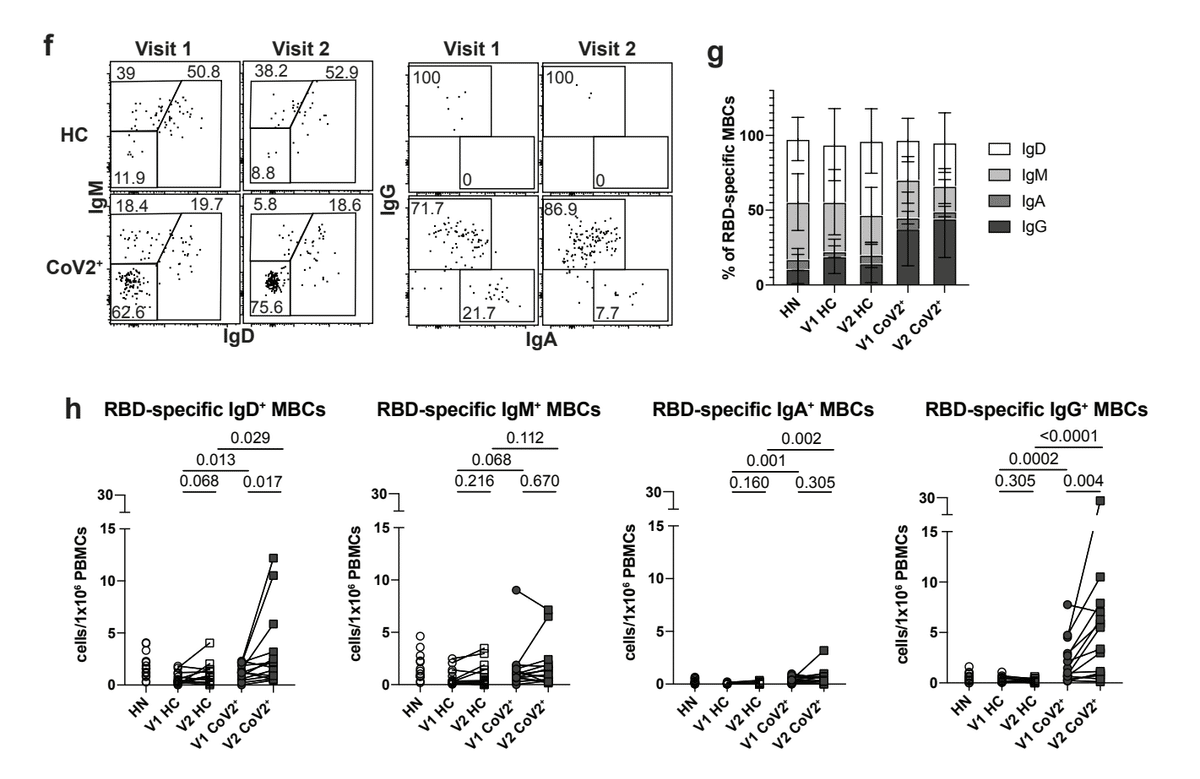
Of note, while small numbers of RBD-specific MBCs were detected in controls, these cells were predominantly unswitched (IgM+ and IgD+), suggesting they may represent cross-reactive MBCs possibly generated in response to one of the human coronaviruses that cause 15% of common colds.
注目すべきことに、少数の RBD 特異的 MBC がコントロールで検出されましたが、これらの細胞は主に切り替えられていません (IgM+ および IgD+)。 これらは、一般的な風邪の 15% を引き起こすヒト コロナウイルスの 1 つに応答して生成された可能性がある交差反応性 MBC を表している可能性があることを示唆しています。
An additional measure of antiviral MBC function is the graded expression of T-bet.
抗ウイルスMBC機能の追加の尺度は、T-betの段階的発現です。
MBCs that express low-levels of T-bet are associated with rapid differentiation into secondary PBs that produce high affinity, viral-specific antibodies during a secondary infection.
低レベルの T-bet を発現する MBC は、二次感染時に高親和性のウイルス特異的抗体を産生する二次 PB への急速な分化と関連しています。
We found a higher proportion and number of T-bet+, and specifically T-betlo, RBD-specific MBCs in CoV2+ individuals compared with HCs at Visit 1 and the higher numbers were maintained at Visit 2 (Fig. 2i-k, E.D. Fig. 5g,h).
訪問1時のHCと比較して、CoV2 +個人ではT-bet +、特にT-betlo、RBD固有のMBCの割合と数が多いことがわかりました。そして、より高い数が訪問 2 で維持された (図 2i-k、ED 図 5g、h)。
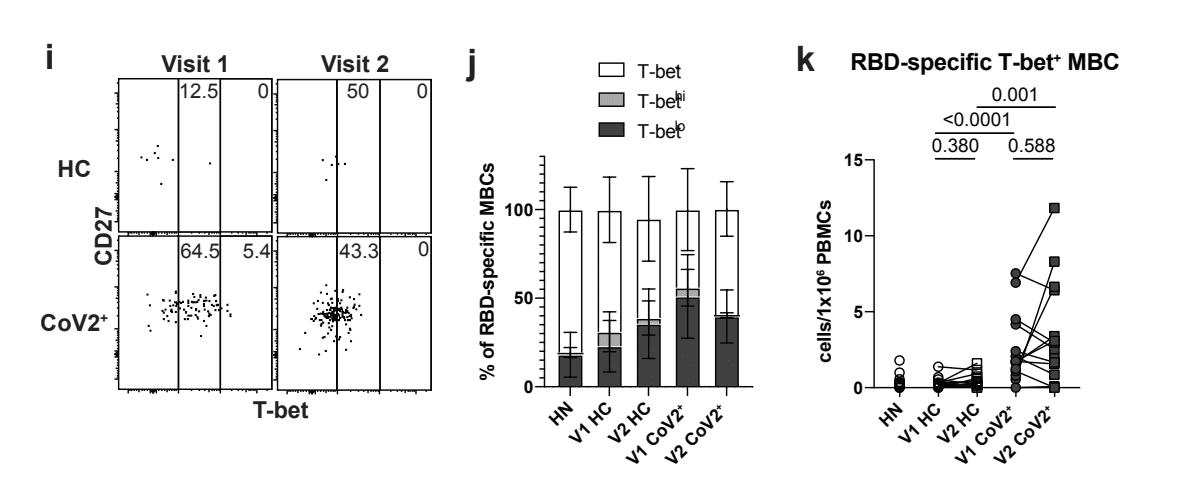
T-bet hi MBCs are considered to be recently activated and often found enriched during chronic infection.
T-bet hi MBCは、最近活性化されたと考えられ、慢性感染中に濃縮されることが多い。
Consistent with SARS-CoV-2 being an acute infection, we found very few RBD-specific T-bethi MBCs in CoV2+ individuals at either memory time point (E.D. Fig. 5i).
SARS-CoV-2が急性感染症であることと一致して、どちらの記憶時点でも、CoV2+個人でRBD特異的なT-bethi MBCがほとんど見つかりませんでした(ED図5i)。
Our data demonstrate that SARS-CoV-2 infection induces the generation of RBD-specific TbetloIgG+CD21+CD27+ “classical” MBCs likely derived from a GC.
私たちのデータは、SARS-CoV-2 感染が RBD 特異的な Tbetlo IgG+CD21+CD27+ の「古典的な」MBC の生成を誘導し、おそらく GC に由来することを示しています。
Furthermore, numbers of these MBCs were not only maintained, but increased from one to three months post-symptom onset.
さらに、これらの MBC の数は維持されただけでなく、発症後 1 か月から 3 か月に増加しました。
SARS-CoV-2 infection induces durable, functional spike-reactive CD4+ T cells
SARS-CoV-2 感染は、耐久性があり機能的なスパイク反応性 CD4+ T 細胞を誘導します
The presence of T-bet+ RBD-specific MBCs suggested that antigen-specific memory T cell responses were also likely to be elicited in CoV2+ individuals.
T-bet+ RBD 特異的 MBC の存在は、抗原特異的メモリー T 細胞応答も CoV2+ 個体で誘発される可能性が高いことを示唆していました。
To enumerate SARS-CoV-2- specific memory T cells, total PBMCs from control or CoV2+ individuals were incubated with spike protein and expression of activation markers was assessed (Fig. 3a).
SARS-CoV-2 特異的記憶 T 細胞を列挙するために、コントロールまたは CoV2+ 個体からの総 PBMC をスパイクタンパク質とインキュベートし、活性化マーカーの発現を評価しました (図 3a)。
PBMCs from CoV2+ individuals at Visit 1 and 2 displayed robust re-activation of spike-specific CD4 + memory T responses, as measured by increased expression of ICOS and CD40L (two molecules associated with B cell help upon re-activation), while PBMCs from HC and HN individuals did not (Fig. 3a,b).
Visit 1 および 2 の CoV2+ 患者の PBMC は、スパイク特異的な CD4 + メモリー T 応答の強力な再活性化を示しました。これは、ICOS および CD40L の発現の増加によって測定されます (B 細胞に関連する 2 つの分子が再活性化に役立ちます)。 一方、HCおよびHN個人のPBMCはそうではありませんでした(図3a、b)。
There were no significant differences in the numbers of responding cells in CoV2+ individuals between the two visits, suggesting spike-specific memory CD4+ T cells were maintained throughout the study (Fig. 3b).
2回の訪問の間でCoV2 +個人の応答細胞数に有意差はなく、スパイク特異的記憶CD4 + T細胞が研究全体で維持されていたことを示唆しています(図3b)。
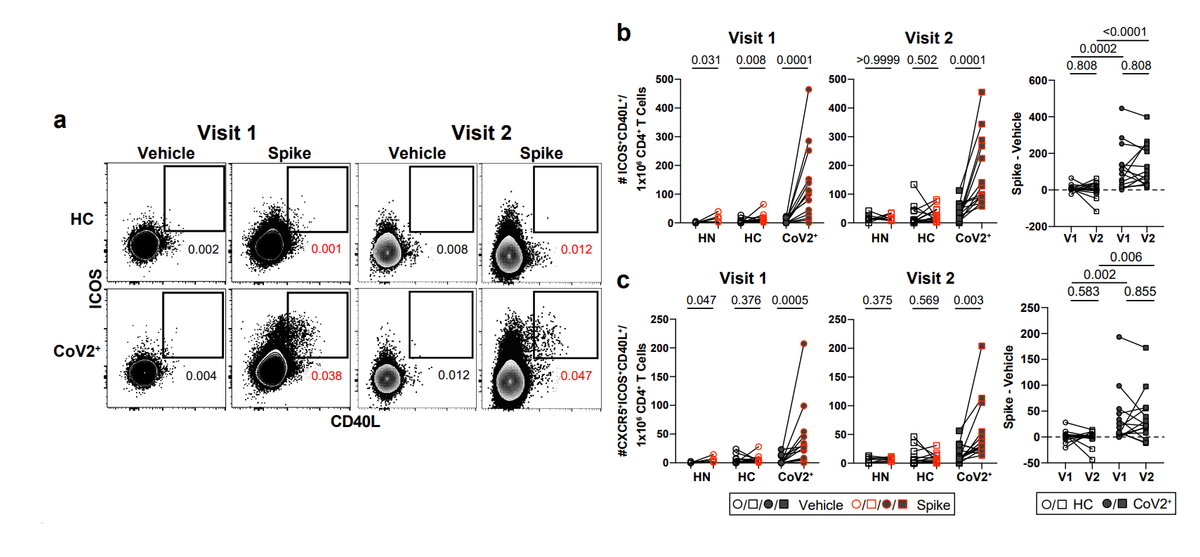
Furthermore, greater numbers of CXCR5-expressing circulating T follicular helper (cTfh) cells, which provide B cell help, were found within the population of S-specific ICOS+CD40L+CD4+ cells in CoV2+ individuals than in healthy controls at both visits (Fig. 3c).
さらに、B細胞に助けを提供するCXCR5発現循環T濾胞性ヘルパー(cTfh)細胞の数が、両方の訪問で健康な対照よりもCoV2 +個人のS特異的ICOS + CD40L + CD4 +細胞の集団内で見つかりました(図 3c)。
Together these data suggest that SARS-CoV-2-specific memory CD4+ T cells maintain the capacity to provide B cell help even at three months post-symptom onset.
これらのデータを総合すると、SARS-CoV-2 特異的記憶 CD4+ T 細胞は、発症から 3 か月後でも B 細胞を助ける能力を維持していることが示唆されます。
Memory CD4+ T cells produce cytokines within hours of activation, whereas naive T cells take days.
メモリー CD4+ T 細胞は活性化から数時間以内にサイトカインを産生しますが、ナイーブ T 細胞は数日かかります。
We first examined cytokine production from activated CD4+ memory CXCR5- non-Tfh cells and CXCR5+ cTfh cells identified in the assay above (Fig. 3b).
最初に、上記のアッセイで同定された活性化 CD4+ メモリー CXCR5- 非 Tfh 細胞および CXCR5+ cTfh 細胞からのサイトカイン産生を調べました (図 3b)。
S-specific CCR6+CXCR5+ cTfh cells, associated with IL-17 production, and a smaller population of CXCR3+CXCR5+ cTfh cells, associated with IFNγ production, were recently described in a predominantly mild to moderate cohort 30 days post symptom onset.
IL-17 産生に関連する S 特異的 CCR6+CXCR5+ cTfh 細胞、および IFNγ 産生に関連する CXCR3+CXCR5+ cTfh 細胞の少数の集団が、発症から 30 日後の主に軽度から中等度のコホートで最近報告されました。
We therefore analyzed activated ICOS+CD69+ S-specific cells for expression of CCR6 and CXCR5 and then cytokine expression was examined in each population based on gating on a PMA positive control (Fig 3d, E.F. 6a).
したがって、活性化されたICOS + CD69 + S特異的細胞をCCR6およびCXCR5の発現について分析し、PMAポジティブコントロールのゲーティングに基づいて各集団でサイトカイン発現を調べました(図3d、EF 6a)。
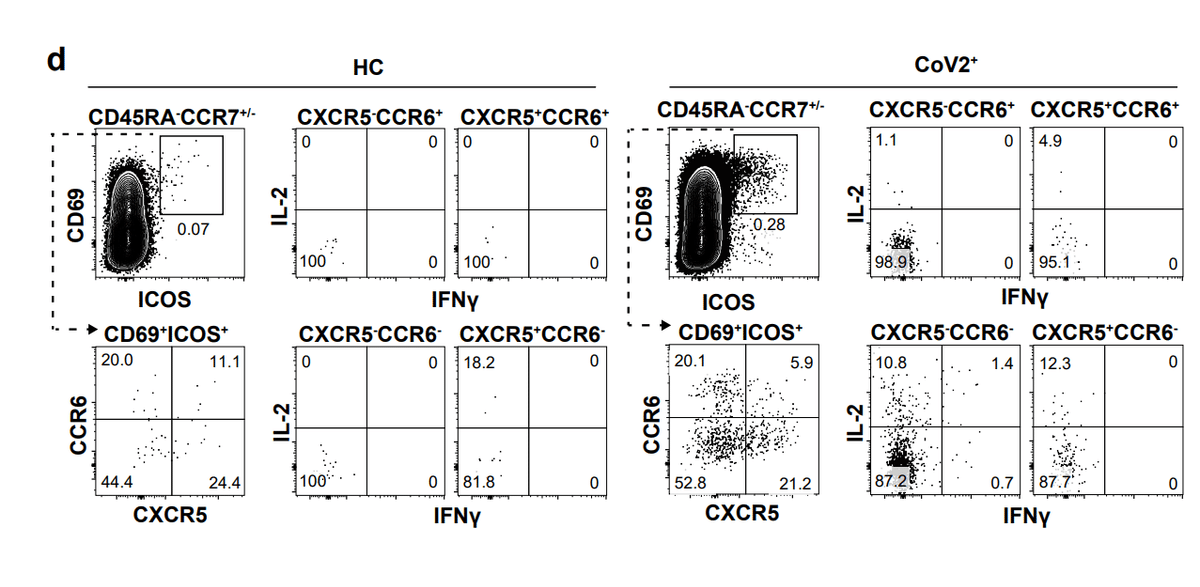
Although multiple cytokines associated with Tfh function were assessed, only IFNγ, IL-17 and IL-2 cytokine producing cells were significantly expressed in activated S-specific memory CD4+ cells in CoV2+ individuals compared to HCs (Fig. 3d-f).
Tfh 機能に関連する複数のサイトカインが評価されましたが、IFNγ、IL-17、および IL-2 サイトカイン産生細胞のみが、HC と比較して CoV2+ 患者の活性化 S 特異的記憶 CD4+ 細胞で有意に発現されました (図 3d-f)。
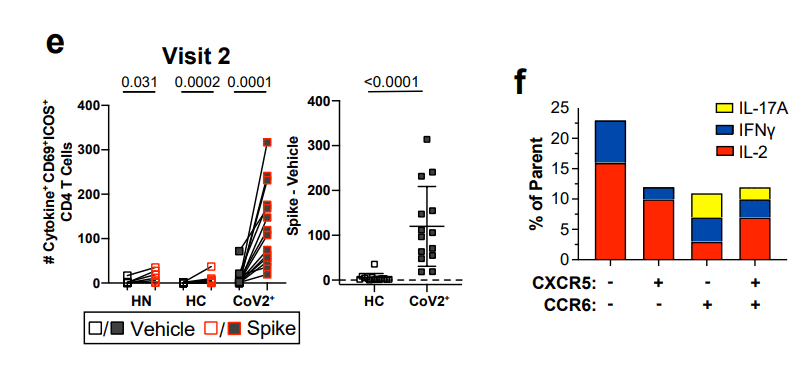
Small numbers of S-specific cells were measured in HCs after stimulation compared to vehicle alone that reflect previously described S-specific cross-reactivity, but far greater responses were seen in the CoV2+ individuals (Fig. 3e).
刺激後の HC では、前述の S 特異的交差反応性を反映するビヒクル単独と比較して少数の S 特異的細胞が測定されましたが、CoV2+ 個体でははるかに大きな応答が見られました (図 3e)。
Three months post symptom onset we found a higher frequency of CCR6- cTfh cells that produced Th1 cytokines, IFNγ and IL-2, suggesting a dominant Th1 response in CoV2+ individuals (Fig. 3f).
症状の発症から 3 か月後、Th1 サイトカインであるIFNγ・IL-2 を産生する CCR6-cTfh 細胞の頻度が高くなり、CoV2+ 患者における優勢な Th1 反応が示唆されました (図 3f)。
To further define the types of antigen-specific CD4+ memory T cells in CoV2+ individuals without relying on secretion of specific cytokines, we assessed memory CD4+ T cell proliferation in response to spike restimulation.
特定のサイトカインの分泌に依存せずに CoV2+ 個体の抗原特異的 CD4+ メモリー T 細胞のタイプをさらに定義するために、スパイク再刺激に応答したメモリー CD4+ T 細胞の増殖を評価しました。
For this, we sorted CD45RA+ naive, CD45RA– CCR7+ central memory (Tcm) and CD45RA– CCR7– effector memory (Tem) T cells from HC or CoV2+ individuals (E.D. Fig. 6b), then measured the proliferative capacity of each sorted population following culture with autologous CD14+ monocytes and recombinant spike protein (Fig. 3g, h; E.D. Fig 6c).
このために、HC または CoV2+ 個体からの CD45RA+ ナイーブ、CD45RA– CCR7+ セントラル メモリー (Tcm) および CD45RA– CCR7– エフェクター メモリー (Tem) T 細胞を選別しました (E.D. 図 6b)。次に、自家CD14 +単球および組換えスパイクタンパク質で培養した後の各選別集団の増殖能を測定しました(図3g、h; E.D.図6c)。
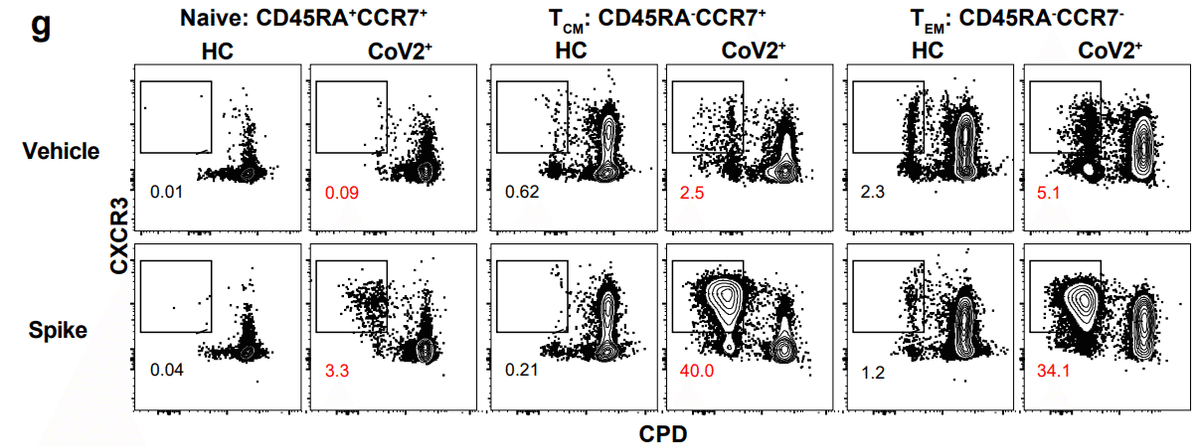
Only Tcm cells from CoV2+ individuals taken at both Visit 1 and Visit 2 displayed significant proliferation frequencies compared to HC samples, although substantial proliferative responses by Tem cells were observed in some CoV2+ individuals (Fig. 3h).
訪問 1 と訪問 2 の両方で採取された CoV2+ 個体からの Tcm 細胞のみが、HC サンプルと比較して有意な増殖頻度を示しましたが、一部の CoV2+ 個体では Tem 細胞による実質的な増殖反応が観察されました (図 3h)。
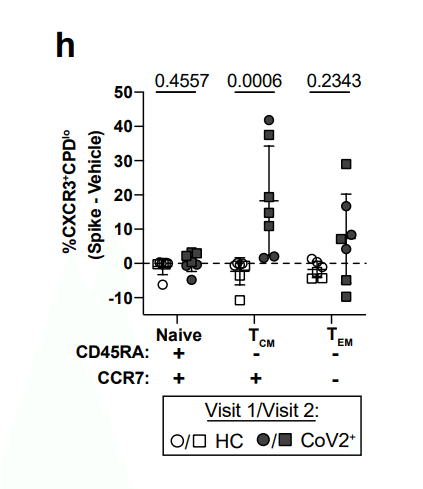
We also examined the expression of CXCR3 and CCR6 on S-specific, proliferated memory cells and found that the majority of cells that had proliferated, as measured by the dilution of cell proliferation dye (CPDlo) expressed CXCR3, in keeping with Type 1 cytokine production in the previous assay.
また、S 特異的な増殖した記憶細胞における CXCR3 および CCR6 の発現も調べました。そして、細胞増殖色素(CPDlo)の希釈によって測定されるように、増殖した細胞の大部分が、以前のアッセイにおけるタイプ1サイトカイン産生と一致して、CXCR3を発現したことを発見しました。
Spike-specific Tcm, and potentially Tem, are therefore maintained throughout our study and have the ability to proliferate and re-populate the memory pool upon antigen re-encounter.
したがって、Spike 固有の Tcm、および潜在的に Tem は、研究全体を通じて維持され、抗原の再遭遇時にメモリ プールを増殖および再生成する能力があります。
While much recent work has focused on antibodies and B cells, memory CD8+ T cells are uniquely positioned to kill virus infected cells through their directed expression of cytokines and cytolytic molecules.
最近の研究の多くは抗体と B 細胞に焦点を当てていますが、メモリー CD8+ T 細胞は、サイトカインと細胞溶解性分子の指示された発現を通じてウイルス感染細胞を殺す独自の位置にあります。
S-specific memory CD8+ T cells that persisted for three months after mild COVID-19 disease could be identified by expression of the activation marker CD69 and the cytokine IFNγ after overnight stimulation with spike (Fig 3i).
軽度の COVID-19 疾患の後 3 か月間持続した S 特異的メモリー CD8+ T 細胞は、スパイクによる一晩の刺激後の活性化マーカー CD69 およびサイトカイン IFNγ の発現によって識別できました (図 3i)。
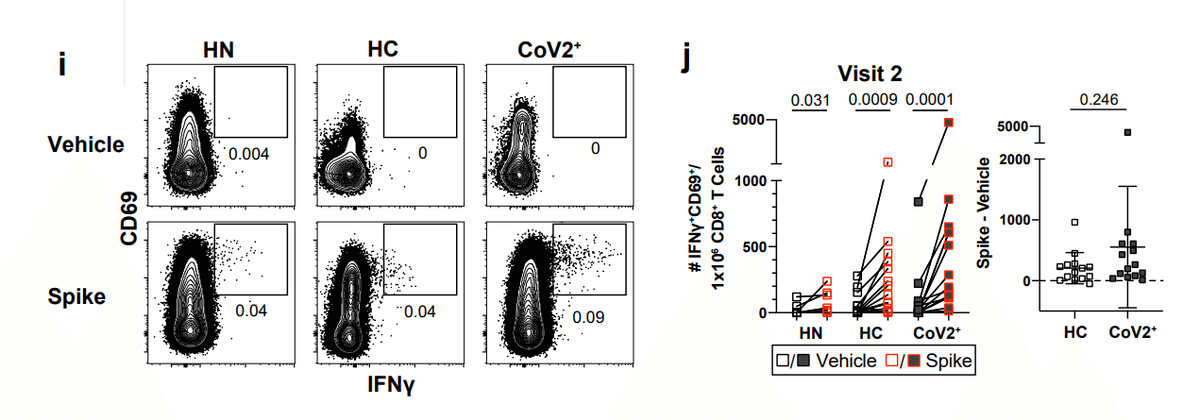
Unlike CD4+ memory T cells, activated cytokine-expressing CD8+ T cells were significantly increased over vehicle controls in both control and CoV2+ groups (Fig. 3j).
CD4+ メモリー T 細胞とは異なり、活性化サイトカイン発現 CD8+ T 細胞は、対照群と CoV2+ 群の両方でビヒクル対照よりも有意に増加しました (図 3j)。
Together, these data demonstrate that both CD4+ and CD8+ SARS-CoV-2-specific memory T cells are maintained and are able to produce effector cytokines after restimulation three months post-symptom onset in mildly symptomatic COVID-19 individuals.
まとめると、これらのデータは、CD4+ と CD8+ の両方の SARS-CoV-2 特異的メモリー T 細胞が維持され、軽度の症状の COVID-19 患者で発症後 3 か月の再刺激後にエフェクター サイトカインを産生できることを示しています。
Mild COVID-19-induced SARS-CoV-2-specific MBCs can express neutralizing antibodies
軽度の COVID-19 誘発 SARS-CoV-2 特異的 MBC は中和抗体を発現できる
Since SARS-CoV-2 RBD-specific MBC and S-specific CD4+ cTfh were enriched in CoV2+ individuals after 3 months, we assessed whether these MBCs could produce neutralizing antibodies if they were reactivated by a secondary infection.
SARS-CoV-2 RBD 特異的 MBC および S 特異的 CD4+ cTfh は 3 か月後に CoV2+ 個体で濃縮されたため、これらの MBC が二次感染によって再活性化された場合に中和抗体を産生できるかどうかを評価しました。
To this end, we index sorted single RBD-specific B cells and sequenced the BCRs from 3 CoV2+ individuals at Visit 1 (E.D. Fig. 7a).
この目的のために、単一の RBD 特異的 B 細胞をインデックスソートし、Visit 1 で 3 人の CoV2+ 個体からの BCR をシーケンスしました (E.D. 図 7a)。
Of the class-switched (IgG+) RBD-specific classical MBCs (CD21+CD27+) we sorted, we randomly selected 7 to be cloned and expressed as IgG1 monoclonal antibodies (Fig 4a).
分類したクラス スイッチ (IgG+) RBD 特異的古典的 MBC (CD21+CD27+) のうち、無作為に 7 つを選択してクローン化し、IgG1 モノクローナル抗体として発現させました (図 4a)。
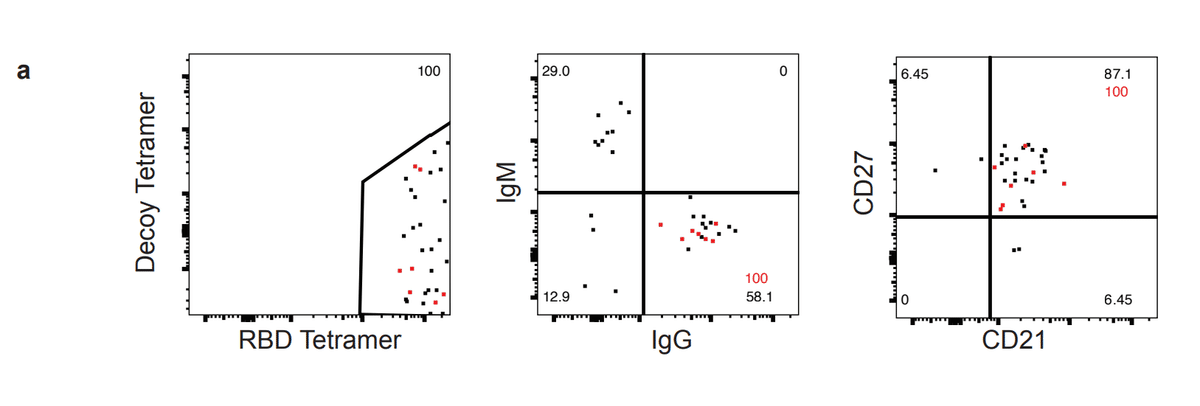
This set of antibodies utilized a wide variety of heavy and light chains, had all undergone somatic hypermutation and were all unique clones (Fig. 4b, E.D. Table 2).
この抗体のセットは、さまざまな重鎖と軽鎖を利用し、すべて体細胞超変異を受けており、すべてユニークなクローンでした(図4b、ED表2)。
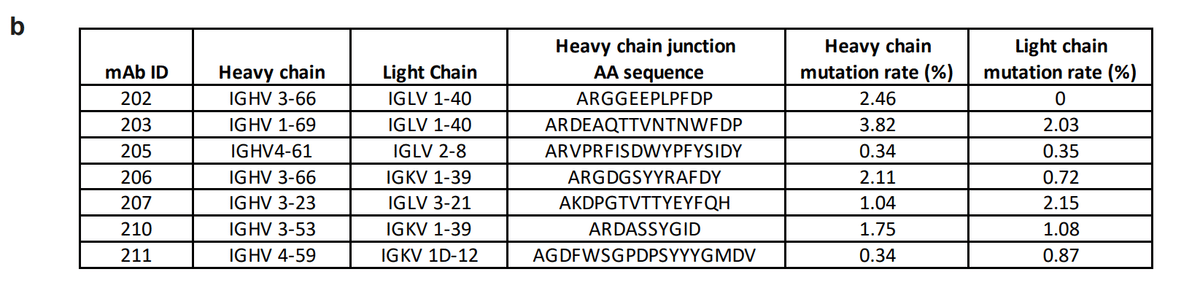
These antibodies were first expressed in small scale cultures.
これらの抗体は、最初に小規模培養で発現されました。
Transfection supernatants were assessed for antibody expression by IgG ELISA (E.D. Fig 7b) and specificity by RBD ELISA where all 7 showed strong binding to RBD (Fig. 4c).
トランスフェクション上清をIgG ELISAによる抗体発現(E.D.図7b)およびRBD ELISAによる特異性について評価し、7つすべてがRBDへの強い結合を示しました(図4c)。
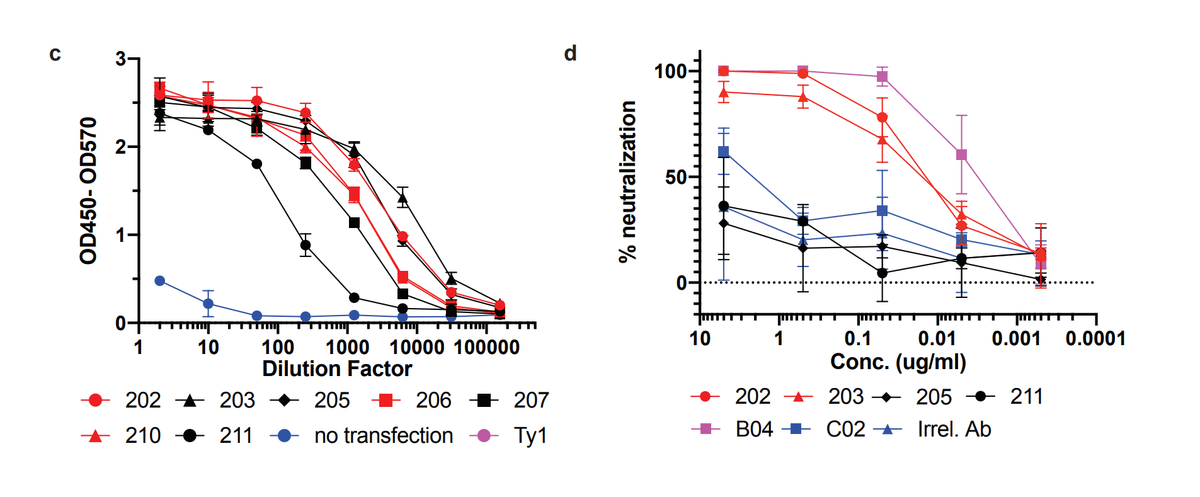
The first 4 antibodies cloned were expressed on a larger scale and purified.
クローン化された最初の4つの抗体は、より大きなスケールで発現され、精製されました。
The specificity of these purified antibodies for RBD was again confirmed by ELISA (E.D. Fig. 7c) and their ability to prevent SARS-CoV-2 infection was tested via PRNT assay.
RBD に対するこれらの精製抗体の特異性は、ELISA によって再度確認され(E.D. 図 7c)、SARS-CoV-2 感染を防ぐ能力は PRNT アッセイによってテストされました。
Two of the four tested (#202 and 203) showed strong virus neutralization (Fig. 4d), with IC50 values of 15.6 and 15.4 ng/ml repectively (Fig. 4e).
テストした 4 つのうちの 2 つ (#202 および 203) は、強力なウイルス中和を示し (図 4d)、IC50 値はそれぞれ 15.6 および 15.4 ng/ml でした (図 4e)。
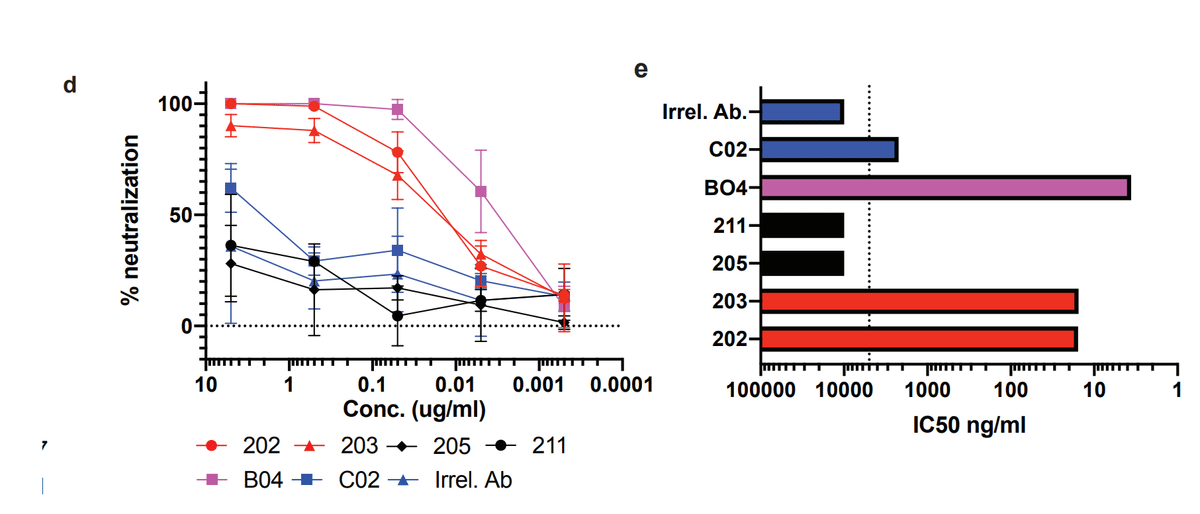
This was comparable to a previously published strongly neutralizing mouse antibody (B04) which was included as a positive control (IC50= 3.6 ng/ml).
これは、陽性対照として含まれていた以前に発表された強力な中和マウス抗体 (B04) に匹敵しました (IC50 = 3.6 ng/ml)。
Two of the RBD-specific antibodies were unable to inhibit virus infection, similar to a non-neutralizing mouse antibody (C02) and an irrelevant Plasmodium-specific human antibody.
RBD 特異的抗体の 2 つは、非中和マウス抗体 (C02) および無関係なマラリア原虫特異的ヒト抗体と同様に、ウイルス感染を阻害できませんでした。
Three more monoclonal antibodies in addition to the 4 above were assessed for their capacity to inhibit RBD binding to the ACE2 receptor by sVNT assay (Fig. 4f).
上記の4つに加えて、さらに3つのモノクローナル抗体を、sVNTアッセイによってACE2受容体へのRBDの結合を阻害する能力について評価しました(図4f)。
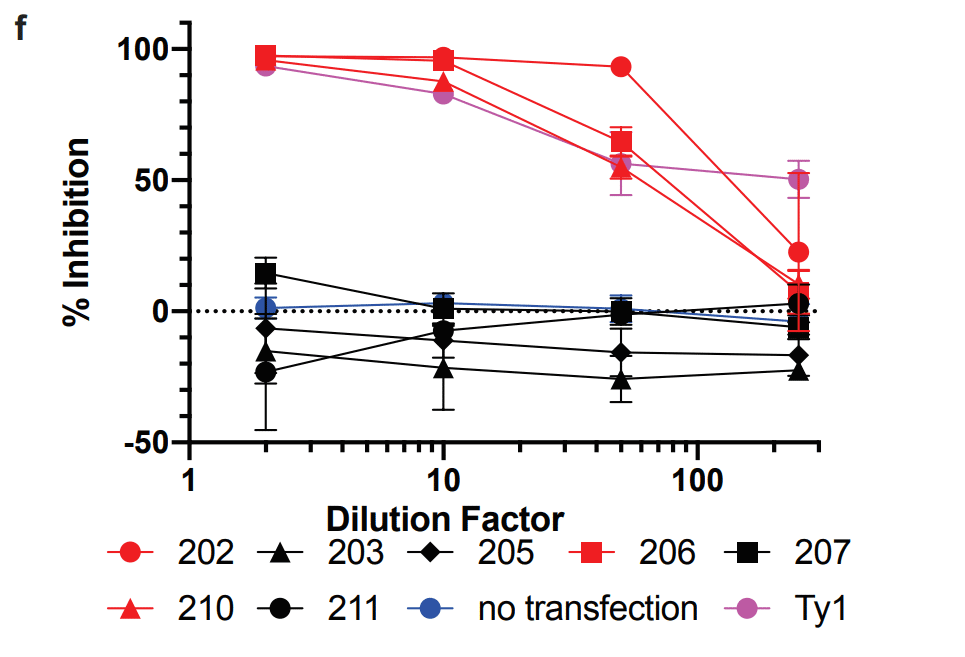
Three of the seven were able to inhibit RBD binding to ACE2, similarly to a strongly neutralizing alpaca nanobody.
7 つのうち 3 つが、強く中和するアルパカ ナノボディと同様に、RBD の ACE2 への結合を阻害することができました。
Interestingly, #203 , which neutralized live virus, did not inhibit binding in this assay, while #202 both inhibited binding and neutralized the virus.
興味深いことに、生きたウイルスを中和した #203 はこのアッセイで結合を阻害しませんでしたが、#202 は結合を阻害し、ウイルスを中和しました。
Overall 50% of the antibodies tested showed inhibitory activity by one or both of these methods.
テストした抗体の全体の 50% が、これらの方法の 1 つまたは両方によって阻害活性を示しました。
Thus, RBD-specific MBCs induced by SARS-CoV-2 infection are capable of producing neutralizing antibodies against the virus and could thus contribute to protection from a second exposure to SARS-CoV-2.
したがって、SARS-CoV-2 感染によって誘導される RBD 特異的 MBC は、ウイルスに対する中和抗体を産生することができ、SARS-CoV-2 への 2 回目の曝露からの保護に寄与する可能性があります。
Discussion
In the absence of a vaccine, natural infection-induced herd immunity could play a key role in reducing infections and deaths.
ワクチンがない場合、自然感染によって誘発される集団免疫は、感染と死亡を減らす上で重要な役割を果たす可能性があります。
For this to be possible, individuals that experience mild COVID-19 would need to develop and sustain protective immune memory.
これが可能になるためには、軽度の COVID-19 を経験した個人は、防御免疫記憶を発達させ、維持する必要があります。
Here, we found that individuals that recovered from mildly symptomatic COVID-19 had an expanded arsenal of SARS-CoV-2- specific immune mediators: neutralizing antibodies, IgG+T-betlo classical MBCs, circulating cytokine-producing CXCR5+ Tfh1 cells, proliferating CXCR3+ CD4 + memory cells and IFNγ producing CD8+ T cells that were maintained to at least three months post-symptom onset.
ここでは、症状が軽度の COVID-19 から回復した個人は、SARS-CoV-2 に固有の免疫メディエーターの武器庫が拡大していることがわかりました: 中和抗体、IgG+T-betlo 古典的 MBC、循環サイトカイン産生 CXCR5+ Tfh1 細胞、増殖中の CXCR3+ CD4 + 記憶細胞、および IFNγ 産生 CD8+ T 細胞は、発症後少なくとも 3 か月間維持されました。
This study predicts that these recovered individuals will be protected from a second SARS-CoV-2 infection and, if so, suggests that Th1 memory should be the target of vaccine elicited memory.
この研究は、これらの回復した個人が 2 度目の SARS-CoV-2 感染から保護されることを予測しており、そうであれば、Th1 記憶がワクチン誘発記憶の標的であることを示唆しています。
Although long-lived immune memory can form to most viruses, some studies examining the longevity of the response to coronaviruses have suggested that this is not the case.
ほとんどのウイルスに対して長寿命の免疫記憶が形成される可能性がありますが、コロナウイルスに対する応答の寿命を調べたいくつかの研究では、そうではないことが示唆されています。
However, more recent studies, including our own, have examined memory time points when only LLPCs, and not short-lived PBs, are producing circulating antibodies.
しかし、私たち自身の研究を含む最近の研究では、短命の PB ではなく LLPC のみが循環抗体を産生している記憶の時点を調べています。
Our study, along with three others clearly demonstrates elevated IgG+ RBD-specific plasma antibodies and neutralizing plasma are generated and maintained for at least 3 months post-SARS-CoV-2 infection.
私たちの研究は、他の3つの研究とともに、上昇したIgG + RBD特異的血漿抗体と中和血漿が生成され、SARS-CoV-2感染後少なくとも3か月間維持されることを明確に示しています。
While antibodies reveal the contributions of LLPCs, functional virus-specific memory B and T cells can also be key to protective immune memory.
抗体が LLPC の寄与を明らかにする一方で、機能的なウイルス特異的記憶 B 細胞および T 細胞も防御免疫記憶の鍵となる可能性があります。
Although previous studies have measured the emergence of SARS-CoV-2-specific MBCs within a month of infection, we characterized SARS-CoV-2-specific MBCs at one and three months from symptom onset.
以前の研究では、感染から 1 か月以内に SARS-CoV-2 固有の MBC の出現が測定されましたが、私たちは発症から 1 か月および 3 か月での SARS-CoV-2 固有の MBC を特徴付けました。
Our study revealed a prominent population of RBD-specific IgG+CD27+CD21+T-bet lo MBCs, which has been associated in other infections with rapid differentiation into antibody-secreting PBs upon re-exposure, effective antiviral responses and long-lived protection.
私たちの研究は、RBD特異的IgG + CD27 + CD21 + T-bet lo MBCの顕著な集団を明らかにしました。これは、他の感染症において、再曝露時の抗体分泌PBへの急速な分化、効果的な抗ウイルス応答 および長寿命の保護と関連しています。
Furthermore, we found some of the RBD-specific MBCs at Visit 1 expressed BCRs capable of neutralizing the virus when expressed as antibodies.
さらに、Visit1 時の RBD 特異的 MBC の一部が、抗体として発現した場合にウイルスを中和できる BCR を発現していることを発見しました。
Since the numbers of these IgG+ RBD-specific MBCs were not only sustained, but continued to increase between one and three months, we predict they are GC-derived.
これらの IgG+ RBD 特異的 MBC の数は維持されただけでなく、1 ~ 3 か月間増加し続けたため、それらは GC 由来であると予測されます。
Thus, MBCs at three months would have undergone increased affinity maturation and we would expect an even higher percentage will be capable of producing neutralizing RBD-specific antibodies upon re-infection.
したがって、3か月のMBCは親和性成熟の増加を受けており、再感染時に中和RBD特異的抗体を産生できる割合がさらに高くなると予想されます。
MBC reactivation requires interactions with memory CD4+T cells, which reactivate MBCs through their expression of key molecules associated with T-B interactions including CXCR5, ICOS, CD40 and a variety of cytokines.
MBC の再活性化には、メモリー CD4+T 細胞との相互作用が必要です。この細胞は、CXCR5、ICOS、CD40、さまざまなサイトカインなど、T-B 相互作用に関連する重要な分子の発現を通じて MBC を再活性化します。
SARS-CoV-2-specific CD4+ memory T cells in recovered individuals exhibited the capacity to express all of these molecules and to undergo robust proliferation upon re-exposure to spike protein.
回復した個人の SARS-CoV-2 特異的 CD4+ メモリー T 細胞は、これらすべての分子を発現し、スパイクタンパク質への再曝露時に強力な増殖を受ける能力を示しました。
Notably, S-specific CD4+ memory T cells from CoV2+ individuals rapidly displayed increased levels of ICOS and CD40L on CXCR5+ and CXCR5– cells after stimulation as well as expression of Th1- and Th17- associated cytokines.
特に、CoV2+ 個体由来の S 特異的 CD4+ メモリー T 細胞は、刺激後、CXCR5+ および CXCR5– 細胞上の ICOS および CD40L レベルの増加と、Th1 および Th17 関連サイトカインの発現を急速に示しました。
These results are consistent with another recent report of SARS-CoV-2- specific cTfh cells, although they detected a high frequency of Th17-like cTfh cells, which could be due to the earlier time point they were examining as Th17 cells can develop into Th1 cells late in an immune response.
これらの結果は、SARS-CoV-2 特異的 cTfh 細胞に関する別の最近の報告と一致していますが、Th17 様 cTfh 細胞が高頻度で検出されました。これは、Th17細胞が免疫応答の後期にTh1細胞に発達する可能性があるため、彼らが調べていたより早い時点が原因である可能性があります。
The expression of IFNγ and IL-17 by these cells is notable as these cytokines are associated with class-switching to IgG and IgA isotypes, respectively.
これらの細胞による IFNγ および IL-17 の発現は、これらのサイトカインがそれぞれ IgG および IgA アイソタイプへのクラス切り替えに関連しているため、注目に値します。
We also found cross-reactive memory B and T cells in healthy controls, as has been previously noted.
また、以前に指摘したように、健康なコントロールで交差反応性のメモリーBおよびT細胞を発見しました。
It is difficult to measure their contribution to the expanded populations of SARS-CoV-2-specific cells we found in our CoV2+ cohorts, and therefore impossible to evaluate their protective capacity.
CoV2+コホートで見つかったSARS-CoV-2特異的細胞の拡大した集団へのそれらの寄与を測定することは困難であり、したがってそれらの保護能力を評価することは不可能です。
However, we can conclude that mild COVID-19 induces an expanded population of functionally diverse memory lymphocytes compared to the cross-reactive pool present in our controls.
ただし、軽度のCOVID-19は、対照に存在する交差反応プールと比較して、機能的に多様な記憶リンパ球の集団の拡大を誘発すると結論付けることができます。
Studies of reinfection have yet to be done in humans, but macaques infected with SARS-CoV-2 were protected from rechallenge.
ヒトでの再感染の研究はまだ行われていませんが、SARS-CoV-2 に感染したマカクは再感染から保護されました。
This further suggests that the immune memory induced by mild COVID-19 that we observed will be protective.
これはさらに、私たちが観察した軽度の COVID-19 によって誘発された免疫記憶が防御的であることを示唆しています。
While additional studies are needed to understand variability of responses in a larger cohort and to determine how long memory to SARS-CoV-2 infection is truly maintained, our work suggests that mild COVID-19 induces persistent immune memory poised for a coordinated, protective response to re-exposure that could contribute to herd immunity and curtailing this pandemic.
より大きなコホートにおける反応の変動性を理解し、SARS-CoV-2 感染に対する記憶が実際にどのくらい維持されるかを判断するには、追加の研究が必要ですが、私たちの研究は、軽度の COVID-19 が持続的な免疫記憶を誘発し、集団免疫に貢献し、このパンデミックを抑えることができる、再曝露に対する調整された防御反応の態勢を整えていることを示唆しています。
以下省略。